the Creative Commons Attribution 4.0 License.
the Creative Commons Attribution 4.0 License.
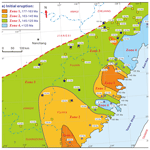
Temporospatial variation in the late Mesozoic volcanism in southeast China
Yongxiang Li
Jingyu Wang
Chaokai Zhang
Yin Wang
Ling Liu
The magmatism (including volcanism) in East Asia (or China) could provide key clues and age constraints for the subduction and dynamical process of the Paleo-Pacific Plate. Although many absolute isotope ages of extrusive rocks have been published in the 1980s–2000s, large uncertainties and large errors prevent the magmatism in southeast (SE) China from being well understood. In this study, we investigate the zircon geochronology of extrusive rocks and temporospatial variations in the late Mesozoic volcanism in SE China. We reported zircon U–Pb ages of new 48 extrusive rock samples in the Shi-Hang tectonic belt. Together with the published data in the past decade, ages of 291 rock samples from ∼40 lithostratigraphic units were compiled, potentially documenting a relatively complete history and spatial distribution of the late Mesozoic volcanism in SE China. The results show that the extrusive rocks spanned ∼95 Myr (177–82 Ma), but dominantly ∼70 Myr (160–90 Ma), within which the volcanism in the early Early Cretaceous (145–125 Ma) was the most intensive and widespread eruption. We propose that these ages represent the intervals of the Yanshanian volcanism in SE China. Spatially, the age geographic pattern of extrusive rocks shows that both the oldest and youngest age clusters occur in the coastal magmatic arc (eastern Zhejiang and Fujian), and the most intensive and widespread age group (145–125 Ma) occurs in a back arc or rifting basin (eastern Jiangxi, central Zhejiang, and northern Guangdong), implying that the late Mesozoic volcanism migrated northwest and subsequently retreated southeast. This volcanic migration pattern may imply that the Paleo-Pacific Plate subducted northwestward and the roll-back subduction did not begin until the Aptian (∼125 Ma) of the mid-Cretaceous.
- Article
(7378 KB) - Full-text XML
-
Supplement
(5968 KB) - BibTeX
- EndNote
It is generally believed that an Andean-type active continental margin developed during the late Mesozoic in eastern Eurasia, along which the Paleo-Pacific Plate (PPP) subducted beneath East Asia (e.g., Taylor and Hayes, 1983; Faure and Natal'in, 1992; Charvet et al., 1994; Zhou and Li, 2000; Chen et al., 2005; Liu et al., 2017; S. Z. Li et al., 2019). The subduction has exerted profound impacts in southeast (SE) China (e.g., Taylor and Hayes, 1983; Zhou and Li, 2000; C. L. Li et al., 2014; J. H. Li et al., 2014; Y. H. Jiang et al., 2015; Liu et al., 2016) and many other parts of East Asia (e.g., Stepashko, 2006; Wu et al., 2007; Choi and Lee, 2011; Zhang et al., 2011; Sun et al., 2013, 2015; Dong et al., 2016; Liu et al., 2017), as indicated by the pervasive crustal deformation associated with the Yanshanian orogeny (e.g., Lapierre et al., 1997; Li, 2000; Zhou and Li, 2000) and the widespread magmatism (e.g., Zhou et al., 2006; Sun et al., 2007). Obviously, the study of the magmatism would help to constrain the process of the PPP subduction.
The late Mesozoic volcanism in SE China, as a response to the PPP subduction, has long attracted attention, and much dating work has been carried out. However, different time intervals and various episodes, cycles, or periods of the volcanism have been proposed (e.g., Li et al., 1989; Feng et al., 1993; Zhang, 1997; Guo et al., 2012; C. L. Li et al., 2014; Liu et al., 2012, 2014, 2016; S. H. Jiang et al., 2015; Ji et al., 2017; Zhang et al., 2018; Yang et al., 2018; Zhang et al., 2019). The issue can be attributed to the following factors: (1) the published ages were generally based on separate and often limited datasets that were commonly from only several to a dozen samples from a local region such as a mining field or a province or at most from a relatively wide area of two neighboring provinces; (2) age data were obtained using different methods, by which the Rb–Sr, K–Ar, and Ar–Ar dating of bulk-dominated samples yielded ages with large uncertainties and large errors in the 1980s–1990s; (3) refined zircon U–Pb ages of the volcanism have not been analyzed for the whole of SE China.
It is essential to obtain spatially more comprehensive datasets from different parts of SE China and also temporally more expanded datasets from sedimentary basin archives that can document the relatively complete volcanic history to achieve a holistic understanding of the late Mesozoic volcanism and geodynamics in SE China.
In this study, we investigate the geochronology of extrusive rocks in the central and northern Shi-Hang tectonic belt (SHTB; e.g., Gilder et al., 1996; Jiang et al., 2011; Yang et al., 2012). The SHTB contains thick sedimentary strata, which are interbedded with extrusive rocks, and thus has the advantage of providing a more complete stratigraphic archive that preserves more complete and recognizable volcanic events. We also compile the published zircon U–Pb isotope geochronological data of extrusive rocks from all of SE China. Obviously, ages of the extrusive rocks can constrain the geochronology of the initiation, evolution, and termination of the late Mesozoic volcanism in SE China. Specifically, we analyze the temporal evolution and the geographical distribution of the late Mesozoic volcanism, which can indirectly help date and better understand the slab subduction between the eastern Asian continent and the western PPP in East Asia (e.g., Gilder et al., 1991, 1996).
The South China Block comprises the Yangtze Block and Cathaysia Block. The Yangtze Block has an Archean to Proterozoic basement, whereas the Cathaysia Block has a Proterozoic basement. Yangtze and Cathaysia blocks amalgamated during the early Neoproterozoic orogeny (e.g., Zhao and Cawood, 1999; Wang et al., 2006; Zheng and Zhang, 2007; Li et al., 2009), forming the Jiangnan orogen. A cover sequence of marine strata from the late Neoproterozoic to the Paleozoic was accumulated on the united South China Block that subsequently underwent the Caledonian orogeny (or the Guangxi movement) in the early Paleozoic (e.g., Guo et al., 1989; Qiu et al., 2000; Charvet et al., 2010) and the Indosinian orogeny in the early Mesozoic (e.g., Carter et al., 2001; Lepvrier et al., 2004).
The major Jiangshan–Shaoxing suture zone separating the Yangtze and Cathaysia blocks (e.g., Jiang et al., 2011; Yang et al., 2012) was reactivated during the Indosinian and Yanshanian movements (e.g., Wang et al., 2013). During the Yanshanian, the Andean-type convergent margin was developed along SE China following the subduction of the PPP (e.g., Taylor and Hayes, 1983; Faure and Natal'in, 1992; Charvet et al., 1994; Zhou and Li, 2000; Chen et al., 2005; Liu et al., 2017; S. Z. Li et al., 2019). A series of NE-striking back arc basins associated with widespread and large-scale magmatism were produced (e.g., Zhou and Li, 2000; Li and Li, 2007; Liu et al., 2014, 2016; Xie et al., 2017; Yang et al., 2017). Since the deposition in these basins was concomitant with volcanism, it is fairly common that the sedimentary successions are interbedded with volcanic rocks. On the basis of the abundance of volcanic rocks in the strata, these basins can be grouped into three types (Fig. 1): volcanic (volcanically-dominated), volcanic–sedimentary, and sedimentary (e.g., Chen et al., 2005; Shu et al., 2009). These three types of basins are roughly separated by two NE-striking fault zones: the Jiangshan–Shaoxing fault zone and the Zhenghe–Dapu fault zone (Fig. 1). The volcanic basins occur SE of the Zhenghe–Dapu fault zone and were formed on the magmatic arc (Lapierre et al., 1997) along the coastline, i.e., the coastal zone (CZ). The volcanic–sedimentary basins occur in the SHTB confined between the two fault zones, and volcanic rocks are typically interbedded and/or intercalated with sedimentary strata, which were constructed in the back arc or rifting basin (e.g., Gilder et al., 1991; Jiang et al., 2009, 2011). Nevertheless, the late Mesozoic volcanic rocks are almost absent west of the Yujiang–Yudu fault zone in sedimentary basins and western SHTB basins (Fig. 1).
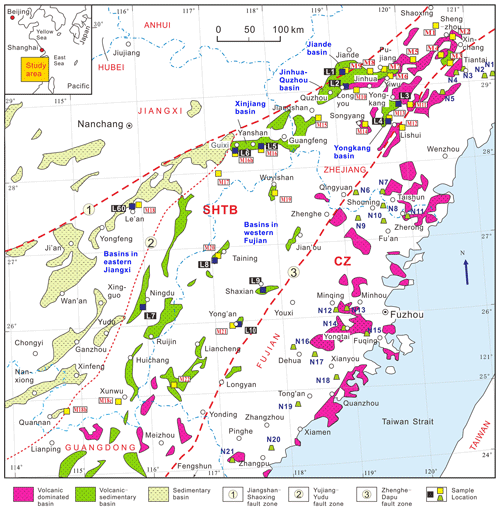
Figure 1Geological map sketch of South China showing tectonic and basin zonations of the upper Mesozoic and sample locations (map simplified after Shu et al., 2009). In SHTB (Shi-Hang tectonic belt), dark blue squares with white capital letter L plus numbers within black rectangles mark the sampling locations of this study (Supplement Figs. S1, S2, and S3), and yellow squares with red capital letter M plus numbers within white rectangles indicate sampling locations of previous studies (Tables S1 and S2 in the Supplement). In CZ (coastal zone), green trapezoids with bold capital letter N plus numbers are sample locations of previous studies (Tables S1 and S3).
The large-scale magmatism is evidenced by the occurrence of granitic plutons in both the SHTB and the CZ stretching over 1000 km along the coastal SE China. These granitic plutons intruded into the Precambrian basement and the overlying Paleozoic strata during the Middle Jurassic–Early Cretaceous (e.g., Jiang et al., 2011; Yang et al., 2012). The intrusions mainly occur as A-type and/or I-type granitic rocks and, together with huge volcanic rocks, strongly support the model of the western subduction of the PPP (e.g., Zhao et al., 2016; Jiang et al., 2011; Yang et al., 2012; Xie et al., 2017; Yang et al., 2017).
A total of 48 extrusive rock samples were collected from about 20 lithostratigraphic formations (Table S1) in 11 basins/regions within the main SHTB to obtain new zircon U–Pb isotope ages (L1–L10 in Fig. 1; Figs. S1–S3 and Table S1). The extrusive rock specimens are volcanic and pyroclastic rocks that are interbedded and intercalated with the sedimentary strata, in which sampling horizons and associated lithologies are marked in Figs. S4–S12. These samples were collected from volcanic layers in the main type sections of typical basins in SE China (Fig. S4–S12). In general, three to four rock samples were taken at the lower (or base), middle, and upper (or top) part when a lithostratigraphic unit has multiple volcanic horizons or a volcanic layer is over 100–200 m thick (see Table S1). The locations of these samples were determined with a GPS device and are marked on the geological maps (Figs. S1–S3 and Table S1).
Zircon grains were separated using the conventional heavy liquid and magnetic techniques. Single zircon grains were handpicked and mounted on adhesive tapes, embedded in epoxy resin, and then polished to about half to one-third of their thickness and photographed in both reflected and transmitted light. Cathodoluminescence (CL) images were taken at the State Key Laboratory for Mineral Deposits Research, School of Earth Sciences and Engineering, Nanjing University, to examine the internal structures of single zircon grains before U–Pb isotope analysis.
LA-ICP-MS (laser ablation inductively coupled plasma mass spectrometry) and U–Th–Pb analyses of single zircon grains were performed on a Nd of YAG 213 laser ablation system (Agilent 7500a, New Wave Research, USA) coupled with a VG PQ Excell ICP-MS (VG PlasmaQuad Excell inductively coupled plasma mass spectrometry), which is housed in the State Key Laboratory for Mineral Deposits Research, Nanjing University. General ablation time is ca. 60 s, and the ablation pit diameter is at 25–35 µm. The ablation repetition rate is 5 Hz with the incident pulse energy of about 10–20 J cm−2. Calibrations of mass fractionation were made using the index sample GEMOC/GJ (608 Ma). In each experiment, a total of 11 to 21 zircon grains were measured, among which 8 to 18 grains yield concordant age data. Prior to each experiment, the standard GJ-1 and Mud Tank samples were measured. Other measurements follow the methods described by Jackson et al. (2004). Analyses of the Mud Tank sample yielded a weighted 206Pb∕238U age of 726±10 to 737±5 Ma (2σ), which is in good agreement with the recommended value (TIMS, thermal ionization mass spectrometry, age Ma, Black and Gulson, 1978).
Data reduction, isotope ratio, age calculation, and Pb correction were conducted with the GLITTER software using zircon 91500 as an external standard. Data processing and plotting were executed with the Isoplot 3.23 programs (Ludwig, 2001). The uncertainties of age results are quoted at a 1σ confidence level, whereas errors for weighted mean ages are quoted at 2σ.
It is worth noting that those aged samples of mafic dykes, basalts, and gabbros were not compiled herein for the analysis of volcanic temporospatial variation in SE China. This is because (1) among the magmatic rocks, gabbros and basalts are rare, and diorites and andesites are even less common in South China (Zhou and Li, 2000), leading to a weak significance in statistics of the volcanic samples; (2) those published ages of the dykes, basalts and gabbros were mainly measured using different (Ar–Ar, K–Ar, Rb–Sr) isotopic methods (e.g., Li, et al., 1989; Chen et al., 2008b; Wang et al., 2008; Meng et al., 2012), likely causing confusion regarding real ages; (3) it is difficult to obtain a good isotopic age for mafic rocks and, in particular, the bulk (basalt) sample ages by K–Ar, Ar–Ar, and Rb–Sr are ∼10–20 Myr younger than those by zircon U–Pb isotopes (Li et al., 2019); and (4) some basalts are predominantly of the Indosinian orogeny age instead of the Yanshanian orogeny.
4.1 Uncertainty of zircon U–Pb ages
It is necessary to first evaluate the uncertainty of the new age results and other cited age data. The uncertainty depends on three aspects, i.e., the origin of zircon, precision, and accuracy (Schoene et al., 2013).
For the origin, all zircons used in this work were microscopically evaluated with CL to ensure that laser ablation positions of zircons are away from the nucleus, cracks, and inclusions. CL images manifest the growth rings. In the concordant 636 zircons of this work, 20 grains (3.1 %) are 0.1–1.0 in Th ∕ U ratio, and 615 (96.7 %) are 1.0–10.0 (Table 1). Th ∕ U ratios of 3539 zircons can be available in the age data from published references. Together with published data and this work, 1766 zircon grains (42.3 %) are 0.1–1.0 in Th ∕ U ratio, 2394 grains (57.3 %) are 1.0–10.0, 14 grains (0.3 %) are > 10.0, and only one is less than 0.1 (Table 1). CL images and Th ∕ U ratios of this work combined the collected data demonstrate that predominant (> 99.9 %), if not all, zircons are magmatic in origin.
Table 1Percentages of single zircons and rock samples in 1σ error (Myr), error ∕ age ratio, and Th ∕ U ratio of the late Mesozoic extrusive rocks in SE China.
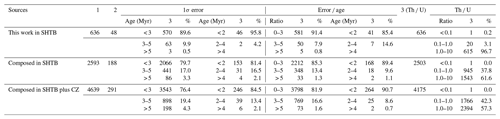
Notes: Numbers of evaluated zircon grains differ from sources in U–Pb age and Th ∕ U ratio due to unavailability of some original data. CZ, coastal zone; SHTB, Shi-Hang tectonic belt. Numbers 1, 2 and 3 in the table stand for the following categories: 1 – concordant zircon number; 2 – rock sample number; 3 – zircon number.
Precision and accuracy uncertainties produced during LA-ICP-MS zircon U–Pb dating have become more and more concerning (e.g., Klötzli et al., 2009; Solari et al., 2010; Li et al., 2015) and come from multiple sources, including the isotopic ratio measurements, the fractionation factor calculation using an external standard, the common lead correction, the external standards, and the data reduction (Li et al., 2015). According to the suggested ∼4 % (2σ) of precision and accuracy (Li et al., 2015), we used the ∼2 % and ∼2 %–4 % (1σ) to evaluate uncertainties of extrusive rock ages.
A total of 48 rock samples were respectively weighted in mean from 636 concordant zircon U–Pb ages in this work (Tables S1 and S2). In the samples, 46 (95.8 %) have a < 3 million years (Myr) error in 1σ; 36 (75 %) samples have < 2 Myr error in 1σ; 41 samples (85.4 %) have < 2.0 % (error ∕ age) deviation, and 7 (14.6 %) have 2 %–4 % deviation (Table 1). Similar percentages of sample error and age deviation are comparable to those single zircons analyzed in this work (Table 1).
For zircons from the published data, the literature often provides CL images of zircons showing quite a similar nature in source and error. For the zircon U–Pb ages from the previous studies, we carefully examine the experiments described in the literature, reanalyze the concordant ages, and eliminate those that are not concordant and/or greater than ∼5 % in age deviation (error ∕ age in percentage) as well as ages with distinct inheritance, which were not discarded by the original authors. This scrutinizing procedure allows us to identify reliable U–Pb age data from 188 volcanic rock samples from the SHTB and from 103 volcanic rock samples from the CZ (Tables S2 and S3). Then, results show that in the combined 291 samples, 246 samples () are < 2 Myr in 1σ error of age and 39 (13.4 %) are 2–4 Myr; 264 samples (90.7 %) are < 2.0 % age deviation and 25 (8.6 %) are 2–4 Myr deviation in age (Table 1). A total of 4639 concordant single zircons are closely similar in percentages of 1σ error and age deviation with the weighted-mean age samples (Table 1).
The above relatively low errors in 1σ and deviation of age indicate that samples of both this and previous work have highly proportional age results (> ∼95 %) with fine precision.
Systematic biases often dominate uncertainty in comparisons between dating methods and between laboratories (Schoene et al., 2013). For measurements of our zircon samples, the internal systematic 2σ error is less than 3 %, which has been verified by reproductive measurements of the Mud Tank sample (see Sect. 3). These systematic biases were mostly met for those zircons from the references. Therefore, small internal systematic 2σ errors allow our zircon date results to be of a moderate accuracy in geochronological application.
The internal systematic conditions are the same for weighted mean dates of individual samples from both this and previous work (refer and compare to Tables S1–S3). Compiled zircons are predominantly single dates generally within less than 2 Myr in 1σ errors (< 3 % biases) for the Late Jurassic–Early Cretaceous volcanic rocks. The dates are to a great degree consistent with the biostratigraphy of pollen spores, plants, ostracods, and conchostracans in the volcanic–sedimentary basins, SHTB (e.g., Chen and Shen, 1982; Sha, 1990; Jiang et al., 1993; Chen, 2000).
In summary, the zircon origin and the age precision and accuracy indicate the sample weighted-mean ages have relatively low uncertainty, and they are suitable for investigating the eruption geochronology of extrusive rocks in SE China.
4.2 U–Pb age spectra of extrusive rocks
Spot analyzing results of this work show that 48 samples have a wide range of (concordant 206Pb∕238U) weighted-mean ages from 162 to 92 Ma (green histogram, Fig. 2a), from which two peaks of weighted mean ages are inconspicuously regressed as 133.3±1.5 and 97.2±1.1 Ma, respectively (Fig. 2a). In addition, 636 concordant single zircons from the samples show a similarly wide age range (166 to 92 Ma) with three age peaks (Fig. 2a, 135.87±0.42, 124.71±0.35, and 98.91±0.57 Ma).
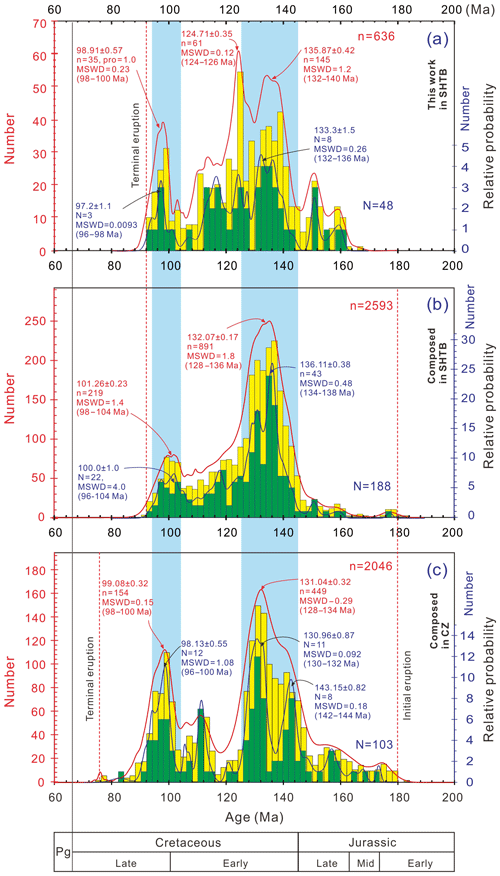
Figure 2Relative probability and histogram diagrams of concordant zircon U–Pb isotope and sample weighted-mean ages of extrusive rocks from SE China (for details, see Tables S1, S2, and S3). (a) This study in SHTB; (b) this and previous studies combined in SHTB; (c) published data in CZ. N: number of rock samples; n: total number of zircon grains.
Combining our new results with the published age data from the main SHTB (e.g., Wu et al., 2011a, b; Wu and Wu, 2013; Liu et al., 2012, 2014, 2016; C. L. Li et al., 2014; J. H. Li et al., 2014; Ma et al., 2016; Wang et al., 2016; Shu et al., 2017; locations M1–M22, see Table S1 and Fig. 1) yields a similar age pattern (Fig. 2b). A total of 188 rock samples show that the weighted-mean ages range from 177 to 92 Ma with a distinct age peak at 136.11±0.38 Ma and an inconspicuous age peak at 100.0±1.0 Ma (Fig. 2b). Also, a total of 2593 single zircons from the SHTB show the concordant 206Pb∕238U ages ranging from 180 to 92 Ma with a strong age peak at 132.07±0.17 Ma and a weak peak at 101.26±0.23 Ma (Fig. 2b).
The published data of 103 rock samples from the CZ (for locations N1–N21 – see Fig. 1 and Tables S1 and S3; Chen et al., 2008a, b; Li et al., 2009; Guo et al., 2012; C. L. Li et al., 2014; Liu et al., 2012, 2016; Zhang et al., 2018) show weighted-mean ages widely ranging from 174 to 82 Ma and three remarkable age peaks of 143.15±0.82, 130.96±0.87, and 98.13±0.55 Ma (Fig. 2c), similar to those from the SHTB (compare to Fig. 2b and c). The 2046 single zircons from the 103 samples also display the same range of concordant 206Pb∕238U ages (Fig. 2c; Table S3) with two prominent age peaks (131.04±0.32 and 99.08±0.32 Ma; Fig. 2c).
Further combined and optimized age data of 291 extrusive rock samples of over 40 lithostratigraphic units in both SHTB and CZ illustrate that sample weighted-mean ages mainly vary between 177 and 82 Ma (Fig. 3). Of the ages, two peaks are at 132.86±0.46 Ma (75 samples, 138–130 Ma; MSWD, mean square weighted deviation, =2.3) and 98.19±0.47 Ma (25 samples, 100–96 Ma; MSWD =1.14), respectively. The compilation of age data from all the 4639 concordant single zircons shows that the 206Pb∕238U ages range between ∼180 and ∼76 Ma with two age peaks at 132.90±0.14 and 99.86±0.19 Ma (Fig. 3).
5.1 Temporal evolution of volcanism
The late Mesozoic extrusive rocks are widespread in SE China and their dating has been conducted extensively. In earlier times, they were roughly dated to the (Late) Jurassic and (Late) Cretaceous by the confinement of interbedded or intercalated terrestrial fossil-bearing sedimentary strata, and the ages are quite crude. Later on, Rb–Sr, K–Ar, and Ar–Ar dating of bulk-dominated samples yielded ages of ∼150–65 Ma with large age uncertainties in the 1980s–1990s (e.g., Hu et al., 1982; Li et al., 1989; Feng et al., 1993; Zhang, 1997), much younger than the earlier rough estimates and ∼10–20 Myr younger than the zircon U–Pb isotope ages on average (Li et al., 2019).
In the recent decade, though zircon U–Pb age data of the igneous rocks have been reported, rock samples in individual references were taken from separate locations resulting in different age interpretations of volcanic eruption in SE China, and a relatively concurrent viewpoint has not been reached. Multiple volcanic age durations are available at different locations or regions, such as 145–129, 143–98, and 140–118 Ma in eastern and northwestern Zhejiang (Liu et al., 2014), 140–88 Ma and 136–129 Ma in southeastern (Liu et al., 2012) and central Zhejiang (J. H. Li et al., 2014), 168–95 Ma in northeastern Guangdong and southeastern Fujian (Guo et al., 2012), 162–130 Ma from two locations in Fujian (Li et al., 2009), 160–99 Ma from northern Fujian (Liu et al., 2016), and 112–99 Ma from the Zijingshan Mineral Field of Fujian (Jiang et al., 2013, 2015). Obviously, these ages are incomplete and intermittent and cannot individually reveal the age of volcanism in all of SE China.
To investigate the geochronology of extrusive rocks, we conducted zircon U–Pb age analysis in the SHTB and combined the published data from both SHTB and CZ. Then, relatively high-precision and representative dating results are obtained in all of SE China: the combined and optimized ages from 291 rock samples (4639 concordant zircons) range from ∼177 to ∼82 Ma (mainly 160–90 Ma).
As we know, the U–Pb isotope ages of zircons represent the ceasing time of the crystalline zircon formation when there is a volcanic eruption; therefore, we propose that the age range above is a suitable representation of the duration of volcanism in SE China. That means, volcanism could have initiated in the late Toarcian (∼177 Ma) of the late Early Jurassic and terminated in the early Campanian (∼82 Ma) of the Late Cretaceous, and it has a ∼95 Myr duration, which shows little discrepancy with those of the single zircon ages (Fig. 3). On the other hand, the volcanism occurred chiefly during the interval of the Late Jurassic–early Late Cretaceous (160–90 Ma; i.e., 70 Myr) when only several samples with ages of pre-160 Ma and post-90 Ma are disregarded (e.g., Chen et al., 2007; Guo et al., 2012; Liu et al., 2012). When considering the relationship of the magmatism to the Yanshanian origin, the above age range and duration (∼177–82 Ma) probably represent the time of the Yanshanian orogeny in East and SE Asia.
Then the temporal evolution scenario of the volcanism in SE China can be summarized as follows (Fig. 3): (1) during the latest Early Jurassic (late Toarcian)–latest Late Jurassic (∼177–145 Ma), the volcanism was sporadic; (2) the early Early Cretaceous (Berriasian–Barremian, ∼145–125 Ma) volcanic eruption was the most intensive; (3) and the volcanism faded during the main mid-Cretaceous (Aptian–Turonian, ∼125–92 Ma); (4) the volcanism almost ceased after that (∼92–82 Ma). The most extensively volcanic eruption episode (145–25 Ma) seems to correspond to the period of rapid increase in the magmatic flux of both the mid-ocean ridge and the large igneous provinces (Coffin and Eldholm, 1994) during the late Late Jurassic–early Early Cretaceous (Fig. 4) although the relationship between them remains unclear.
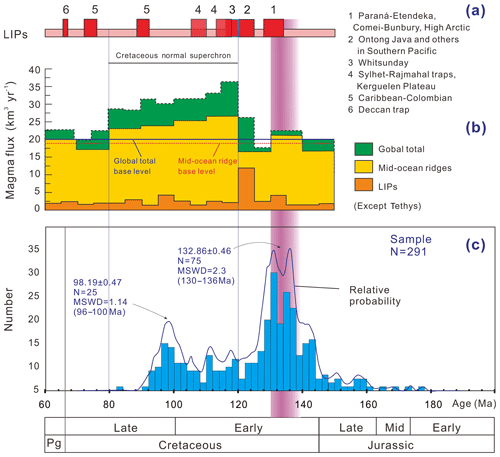
Figure 4Diagram showing age ranges of volcanism in SE China and correlations with the global large igneous provinces (LIPs) and magmatic flux. (a) Age range of the Cretaceous LIPs (for a summary, see Coffin and Eldholm, 1994); (b) magma flux of the Cretaceous LIPs, mid-ocean ridges, and (except Tethys) global total (Coffin and Eldholm, 1994); (c) age range of the volcanism with histogram and relative probability in SE China.
It is noted that among the compiled single zircon U–Pb ages of extrusive rocks, the oldest one is from the Maonong Formation in the Songyang Basin, southwestern Zhejiang. The weighted mean age is 177.4±1.0 Ma for the sample MN01 (location M14; Liu et al., 2012). In addition, a weighted mean age of 180±4 Ma from the same horizon (Chen et al., 2007) has also been reported despite that the error being relatively large (up to 6–8 Myr).
Similarly, variable youngest ages of volcanic rocks are reported. The weighted-mean age 82.5±1.0 Ma of the sample ZJ23 (location N2; Chen et al., 2008a) from the Taozu section of eastern Zhejiang could be the youngest age. One zircon grain from the section is dated to 74±0.6 Ma and five zircon grains yield concordant ages of 76±0.6 Ma from the same sample (Table S3, Chen et al., 2008a), suggesting that it is possible that the termination of volcanism was ∼5 Myr later than 82.5 Ma.
Two hiatuses in volcanism at 128–122 and 120–110 Ma were recently proposed in eastern Zhejiang (Liu et al., 2012), and volcanic reticence at 130–115 Ma was reported in northeastern Guangdong and southeastern Fujian (Guo et al., 2012; N17, N19, N20 in Fig. 1). Similar silence or inactivity of volcanism seems to have occurred in other parts of SE China. However, this volcanic silence seems misleading because it would not appear if we saw all of the late Mesozoic volcanism in SE China.
5.2 Spatial pattern of volcanism
Though it is well-known that late Mesozoic magmatic rocks are widespread in SE China, the previous volcanic distributions are to some degree out of date as those ages contain large errors with low preciseness and accuracy by bulk isotope dating (e.g., Li et al., 1989; Wang et al., 2000; Zhou and Li, 2000; Chen et al., 2008b) and detailed age distribution patterns by precise age constraints have not been outlined yet. To delineate the spatial variation in the late Mesozoic volcanism in SE China that is refined by the zircon U–Pb geochronology, we sketched three distribution maps of extrusive rocks by the initial, peak, and terminal ages of volcanism (Fig. 5a, b, and c).
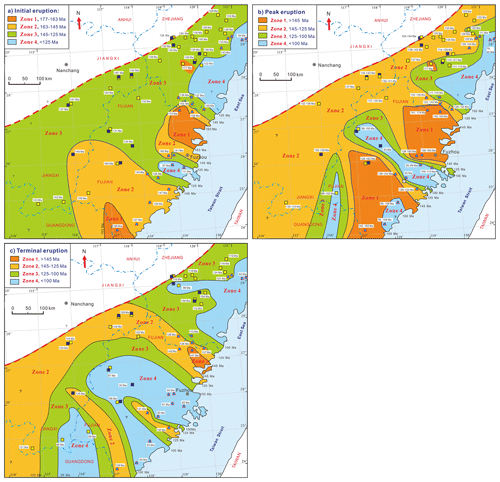
Figure 5Sketch maps showing zonations of the late Mesozoic volcanism by age in SE China (map modified from Shu et al., 2009). Age within white rectangles is the eruption time at a location or in a basin or region. Names of color squares and trapezoids refer to Fig. 1. (a) Zonations of initial eruption ages; (b) zonations of peak eruption ages; (c) zonations of terminal eruption ages.
Firstly, we identified the initial ages of extrusive rocks. The initial age is defined as the earliest age of volcanic eruption in a location, a basin, and/or a region marked by the capital letters L, M, and N with numbers in Fig. 1. Three age boundaries (∼163, ∼145, and ∼125 Ma) are chosen to divide the initial ages into four intervals: 177–163, 163–145, 145–125, and < 125 (−94) Ma. These ordinarily correspond to the epochs of the Middle and Late Jurassic and the early and late Early Cretaceous, respectively. We used the boundary age 177 Ma as the earliest boundary within the first period of the volcanism because it could represent the initiation time of the first Yanshanian orogenic episode in SE China and the corresponding stratal boundary is marked by an unconformity (e.g., Yu et al., 2003; Shu et al., 2009). The boundary between the Upper Jurassic and the Lower Cretaceous is also represented by a widely observed unconformity (e.g., Yu et al., 2003; Shu et al., 2009) and the intensification of volcanism in SE China (Fig. 3). As there are fewer samples with ages of < 125 Ma and the age boundary at ∼125 Ma marks the rapid waning of volcanism (Fig. 3), we designed the interval 125–94 Ma of volcanism as the latest initial age recognition.
Then, isoline ages are drawn by the boundary ages 163, 145, and 125 Ma, separately. Interpolation ages are used to confine the zones when there are no ages exactly the same as the boundary age occurring on the map. Plotting the initial ages in the geographical map shows four zones of initial volcanism in SE China (Fig. 5a). Zone 1 (Middle Jurassic, 177–163 Ma) marks areas where initial volcanic eruption occurs locally in the northernmost corner of Guangdong and the neighboring southern corner of Fujian as well as the northeastern corner of Fujian and at one location of southwestern Zhejiang (M14, Songyang, Liu et al., 2012). Zone 2 (Late Jurassic, 163–145 Ma) delineates areas where initial volcanic eruption occurs around Zone 1 in southern and northeastern Fujian with a much larger scope in southern Fujian than Zone 1. Zone 3 (early Early Cretaceous, 145–125 Ma) defines regions where initial volcanic eruption chiefly and largely extends into SE China and is mostly bounded in the west of the volcanic area, extending along eastern Jiangxi, northwestern Fujian, and central Zhejiang (Fig. 5a). Zone 4 (late Early Cretaceous, 125–94 Ma) locally occupies eastern Zhejiang and, in a limited way, southeastern Fujian (south of Fuzhou). Same zones can be also recognized on the map made from the single zircon U–Pb ages (compare to Fig. S13), supporting the zonations of the sample weighted-mean ages.
Secondly, the peak eruption age of extrusive rocks can be identified, which is defined as the main age of extensively volcanic eruption in a location, a basin, and/or a region marked with L, M, and N with numbers in Fig. 1. Here we use 145, 125, and 100 Ma as three boundary ages to identify the most extensive volcanism in SE China. This is because the main ages are much younger than 145 Ma and few samples show ages younger than 100 Ma, for which the main age isolines are more readily made. Similarly, the corresponding age intervals confined by the boundary ages pertain to the epochs of the Late Jurassic, the early and late Early Cretaceous, and the early Late Cretaceous, respectively.
Isolines of boundary ages are delineated by 145, 125, and 100 Ma and completed with interpolation ages when there are no exact ages in the transition zone. Four zones of peak volcanism are then shown in the geographical map of SE China (Fig. 5b). Zone 1 (Late Jurassic, 163–145 Ma) is the area where most intensive volcanic eruption occurred in southeastern and northeastern Fujian and locally at a place in southwestern Zhejiang (Fig. 5b). Zone 2 (early Early Cretaceous, 145–125 Ma) largely extends along eastern Jiangxi, central Zhejiang, northwestern Fujian, and northern Guangdong (Fig. 5b) and indicates widespread volcanism in SE China. Zone 3 (late Early Cretaceous, 125–100 Ma) occurs as a band in central Zhejiang, southern Fujian, and northeastern Guangdong. Zone 4 (early Late Cretaceous, 100–76 Ma) is locally distributed along Zone 3.
Thirdly, we use the terminal eruption age of extrusive rocks to represent the termination time of the last volcanism, which is helpful to distinguish the terminal volcanic distribution in SE China. The age boundaries and intervals are the same as the peak eruption. It is noted that only one age is older than 145 Ma in the northeastern corner of Fujian and many samples are younger than 100 Ma.
With the confinement of boundary ages 145, 125, and 100 Ma, age isolines are drawn separately, and the interpolation method was used to define the zones when there are no exact ages on the map. Four age zones of the terminal volcanism are recognized on the geographical map by the age isolines (Fig. 5c). Zone 1 (> 145 Ma) occurs in the northeastern corner of Fujian due to only one location of the terminal age. Zone 2 (145–125 Ma) mainly occurs in eastern Jiangxi and the banded boundary of northern Fujian; Zone 3 (125–100 Ma) is largely distributed in the boundary region of eastern Jiangxi and western Fujian and in central and southwestern Zhejiang. Zone 4 (100–83 Ma) appears widely in regions of central Fujian, eastern Zhejiang, and northern Guangdong. Similar zonations can be classified on the map sketched by the single zircon U–Pb ages (Fig. S14), verifying the zones of the sample weighted-mean ages in SE China.
Zonations of initial, peak, and terminal volcanism indicate a distinct pattern of volcanic extrusion in SE China (Fig. 5): the oldest ages in eastern SE China, the younger intensive age clusters in western SE China, and the youngest ones in eastern SE China again. Detailed distributional patterns can be observed: (1) the earliest appearance and earliest disappearance of extrusive rocks dominantly occur in southeastern and northeastern Fujian, where the magmatic arc was located (e.g., Lapierre et al., 1997); (2) the most widespread distribution of extrusive rocks is the most intensive volcanism age (145–125 Ma in eastern Jiangxi, central Zhejiang, and northern Guangdong), in which a back arc or rifting basin was developed (e.g., Gilder et al., 1991; Jiang et al., 2009; 2011); (3) the latest appearance and latest disappearance mainly occur in eastern Zhejiang, eastern Fujian, and northern Guangdong.
With the observation of volcanism, two distributional patterns become manifest: (1) the migration of the volcanism was from the northwest to the southeast, implying that the PPP could have been subducted northwest during the late Mesozoic time; (2) the first appearance (initial volcanism) area and the first disappearance (terminal volcanism) region are the same, suggesting that a roll-back subduction of the PPP happened after ∼125 Ma.
It is surprising that the zones 1 and/or 2 of volcanism look like thermal-dome patterns (Fig. 5) by exhumation and exposure that may be related to the regional magmatic intrusion, likely providing misleading conclusions on the migration of volcanism. However, the distribution pattern is not dome-controlled because (1) the data are derived from extrusive rocks, instead of intrusive rocks; (2) it is impossible that a crater is over 200–300 km wide in diameter; (3) many agglomerates representing craters were observed in a variety of strata at locations or basins outside of Zone 1. For instance, these agglomerates are widespread in basins of western Zhejiang (L1–L4; M9–M14), eastern Jiangxi (L5–L7; M16–M18b), and western Fujian (L8–L10; M19–M22).
We analyzed weighted mean ages of 48 extrusive rock samples (a total of 636 concordant single zircons) from ∼20 lithostratigraphic units at 11 localities in the SHTB. Published ages of 243 rock samples (total of 4003 concordant zircons) from ∼40 lithostratigraphic units in SE China are compiled and reexamined. Based on a total of 291 refined sample ages (4639 concordant zircon U–Pb ages) from this study and the published literature, we propose that the late Mesozoic volcanism in SE China initiated at ∼177 Ma (late Toarcian of the late Early Jurassic) and terminated at ∼82 Ma (early Campanian of the Late Cretaceous), spanning a ∼95 Myr interval (mainly ∼70 Myr =160–90 Ma), during which the 145–125 Ma (the early Early Cretaceous) volcanism is the most intensive and widespread magmatic eruption. This age range and span may represent the time of the Yanshanian magmatism in SE China.
Isolines of initial, peak, and terminal volcanic ages are drawn to outline the geographic distribution of extrusive rocks in SE China. The volcanic extrusion age spatial change shows a distinct pattern of the late Mesozoic volcanism: both the oldest and youngest ages occur in eastern (coastal) Zhejiang and Fujian (magmatic arc); the most intensive and widespread ages are distributed in eastern Jiangxi, middle Zhejiang, and northern Guangdong (back arc/rifting basin), which are located hundreds of kilometers away from the coastline. The geographical distribution pattern of the volcanic eruption ages indicates a migration process of magmatic extrusion in SE China and implies that a northwestern subduction of the Paleo-Pacific Plate happened and a possible roll-back subduction did not begin until the Aptian (∼125 Ma) of the mid-Cretaceous.
All the data are available in the Supplement.
The supplement related to this article is available online at: https://doi.org/10.5194/se-10-2089-2019-supplement.
XL designed the concept and working idea, took samples, and created the paper text, tables, and figures; YL took part in some of the field work and contributed to writing the paper and polishing the language; JW and CZ took part in field work, measured the zircon U–Pb isotopes, and compiled published age data; YW and LL took part in field work and measured the zircon U–Pb isotopes of some samples.
The authors declare that they have no conflict of interest.
We thank Ke Cao, Sijing Liang, Yannan Ji, and Sihe Wang for participating in the field investigation. We are grateful to the reviewers for their helpful comments and constructive suggestions.
This research has been supported by the National Key R&D Plan (grant no. 2017YFC06014005), Natural Science Foundation of China (NSFC) projects 41372106 and 41672097, and National Basic Research Program of China (973 Project) 2012CB822003.
This paper was edited by Mark Allen and reviewed by two anonymous referees.
Black, L. P. and Gulson, B. L.: The age of the Mud Tank carbonatite, Strangways Range, Northern Territory: BMR, J. Aust. Geol. Geophy., 3, 227–232, 1978.
Carter, A., Roques, D., and Bristow, C.: Understanding Mesozoic accretion in Southeast Asia: Significance of Triassic thermotectonism (Indosinian orogeny) in Vietnam, Geology, 29, 211–214, 2001.
Charvet, J., Lapierre, H., and Yu, Y. W.: Geodynamic significance of the Mesozoic volcanism of southeastern China, J. SE Asi. Ear. Sci., 9, 387–396, 1994.
Charvet, J., Shu, L. S., Faure, M., Choulet, F., Wang, B., Lu, H. F., and Le Breton, N.: Structural development of the Lower Paleozoic belt of South China: genesis of an intracontinental orogen, J. Asian Earth Sci., 39, 309–330, 2010.
Chen, C. H., Lin, W., Lan, C. Y., and Lee, C. Y.: Geochemical, Sr and Nd isotopic characteristics and tectonic implications for three stages of igneous rock in the late Yanshanian (Cretaceous) orogeny, SE China, Geol. Soc. Am. Spec. Pap., 389, 237–248, 2005.
Chen, C. H., Lee, C. Y., Lu, H. Y., and Hseh, P. S.: Generation of Late Cretaceous silicic rocks in SE China: Age, major element and numerical simulation constraints, J. Asian Earth Sci., 31, 479–498, 2008a.
Chen, C. H., Lee, C. Y., and Shinjo, R.: Was there Jurassic paleo-pacific subduction in South China?: constraints from 40Ar-39Ar dating, elemental and Sr-Nd-Pb isotopic geochemistry of the Mesozoic basalts, Lithos, 106, 83–92, 2008b.
Chen, P. J.: The classification and correlation of non-marine Jurassic and Cretaceous of China: Comment, J. Stratig., 24, 114–119, 2000 (in Chinese with English abstract).
Chen, P. J. and Shen, Y. B.: The Late Mesozoic conchostracan fossils in Jiangsu, Zhejiang and Anhui provinces, Palaeont. Sin. China, 161, 2–27, 1982 (in Chinese with English abstract).
Chen, R., Xing, G. F., Yang, Z. L., Zhou, Y. Z., Yu, M. G., and Li, L. M.: Early Jurassic zircon SHRIMP U-Pb age of the dacitic volcanic rocks in the southeastern Zhejiang Province determined firstly and its geological significances, Geol. Rev., 53, 31–35, 2007 (in Chinese with English abstract).
Chen, W. F., Chen, P. R., Xu, X. S., and Zhang, M.: Geochemical characteristics of Cretaceous basaltic rocks in South China and constraints on Pacific Plate subduction, Sci. China (Series D), 48, 2104–2117, 2005.
Choi, T. and Lee, Y. I.: Thermal histories of Cretaceous basins in Korea: Implications for response of the East Asian continental margin to subduction of the Paleo-Pacific Plate, Isl. Arc., 20, 371–385, 2011.
Coffin, M. F. and Eldholm, O.: Large igneous provinces: Crustal structure, dimensions, and external consequences, Rev. Geophys., 32, 1–36, 1994.
Dong, Y., Ge, W. C., Yang, H., Xu, W. L., Zhang, Y. L., Bi, J. H., and Liu, X. W.: Geochronology, geochemistry, and Hf isotopes of Jurassic intermediate acidic intrusions in the Xing'an Block, northeastern China: Petrogenesis and implications for subduction of the Paleo-Pacific oceanic plate, J. Asian Earth Sci., 118, 11–31, 2016.
Faure, M. and Natal'in, B.: The geodynamic evolution of the eastern Eurasian margin in Mesozoic times, Tectonophysics, 208, 397–411, https://doi.org/10.1016/0040-1951(92)90437-B, 1992.
Feng, Z. Z.: Mesozoic volcanism and tectonic environments in Fujian, Reg. Geol. China, 4, 311–316, 1993 (in Chinese with English abstract).
Gilder, S. A., Keller, G. R., and Luo, M.: Eastern Asia and the western Pacific timing and spatial distribution of rifting in China, Tectonophysics, 197, 225–243, 1991.
Gilder, S. A., Gill, J., and Coe, R. S.: Isotopic and paleomagnetic constrains on the Mesozoic tectonic evolution of South China, J. Geophys. Res., 101, 16137–16154, 1996.
Guo, F., Fan, W., Li, C., Zhao, L., Li, H., and Yang, J.: Multi-stage crust–mantle interaction in SE China: temporal, thermal and compositional constraints from the Mesozoic felsic volcanic rocks in eastern Guangdong-Fujian provinces, Lithos, 150, 62–84, 2012.
Guo, L. Z., Shi, Y. S., Lu, H. F., Ma, R. S., Dong, H. G., and Yang, S. F.: The pre-Devonian tectonic patterns and evolution of South China, J. SE Asi. Ear. Sci., 3, 87–93, 1989.
Hu, H. G., Hu, S. L., Wang, S. S., and Zhu, M. The Jurassic and Cretaceous age of volcanic rocks on isotope dating, Act Geol. Sin., 56, 315–322, 1982 (in Chinese with English abstract).
Jackson, S. E., Pearson, N. J., Griffin, W. L., and Belousova, E. A.: The application of laser ablation-inductively coupled plasma–mass spectrometry to in situ U/Pb zircon geochronology, Chem. Geol., 211, 47–69, 2004.
Ji, W. B., Lin, W., Faure, M., Chen, Y., Chu, Y., Xu, Z. H.: Origin of the Late Jurassic to Early Cretaceous peraluminous granitoids in the northeastern Hunan province (middle Yangtze region), South China: Geodynamic implications for the Paleo-Pacific subduction, J. Asian Earth Sci., 141, 174–193, 2017.
Jiang, S. H., Liang, Q. L., Bagas, L., Wang, S. H., Nie, F. J., and Liu, Y. F.: Geodynamic setting of the Zijinshan porphyry-epithermal Cu-Au-Mo-Ag ore system, SW Fujian Province, China: constrains from the geochronology and geochemistry of the igneous rocks, Ore Geol. Rev., 53, 287–305, 2013.
Jiang, S. H., Bagas, L., and Liang, Q. L.: New insights into the petrogenesis of volcanic rocks in the Shanghang Basin in the Fujian Province, China, J. Asian Earth Sci., 105, 48–67, 2015.
Jiang, W. S., Zhen, J. S., Li, L. T., and Xu, K. D.: Study of the Cretaceous in Zhejiang, China, Nanjing, Nanjing University Press, 1–42, 1993 (in Chinese with English summary).
Jiang, Y. H., Jiang, S. Y., Dai, B. Z., Liao, S. Y., Zhao, K. D., and Ling, H. F.: Middle to Late Jurassic felsic and mafic magmatism in southern Hunan Province, Southeast China: implications for a continental arc to rifting, Lithos, 107, 185–204, 2009.
Jiang, Y. H., Zhao, P., Zhou, Q., Liao, S. Y., and Jin, G. D.: Petrogenesis and tectonic implications of Early Cretaceous S-and A-type granites in the northwest of the Gan-Hang rift, SE China, Lithos, 121, 55–73, 2011.
Jiang, Y. H., Wang, G. C., Liu, Z., Ni, C. Y., Qing, L., and Zhang, Q.: Repeated slab advance-retreat of the Palaeo-Pacific plate underneath SE China, Int. Geol. Rev., 57, 472–491, 2015.
Klötzli, U., Klötzli, E., Günes, Z., and Kosler, J.: Accuracy of laser ablation U-Pb zircon dating: Results from a test using five different reference zircons, Geostand. Geoanal. Res., 33, 5–15, 2009.
Lapierre, H., Jahn, B. M., Charvet, J., and Yu, Y. W.: Mesozoic felsic arc magmatism and continental olivine tholeiites in Zhejiang Province and their relationship with tectonic activity in SE China, Tectonophysics, 274, 321–338, 1997.
Lepvrier, C., Maluski, H., Maluski, H., Van Tich, V., Leyreloup, A., Thi, P. T., and Van Vuong, N.: The Early Triassic Indosinian orogeny in Vietnam (Truong Son Belt and Kontum Massif); implications for the geodynamic evolution of Indochina, Tectonophysics, 393, 87–118, 2004.
Li, C. L., Wang, Z. X., Wang, D. X., Cao, W. T., Yu, X. Q., Zhou, G. Z., and Gao, W. L.: Crust-mantle interaction triggered by oblique subduction of the Pacific plate: geochronological, geochemical, and Hf isotopic evidence from the Early Cretaceous volcanic rocks of Zhejiang Province, southeast China, Int. Geol. Rev., 56, 1732–1753, https://doi.org/10.1080/00206814.2014.956347, 2014.
Li, J. H., Ma, Z. L., Zhang, Y. Q., Dong, S. W., Li, Y., Lu, M. A., and Tan, J. Q.: Tectonic evolution of Cretaceous extensional basins in Zhejiang Province, eastern South China: Structural and geochronological constraints, Int. Geol. Rev., 56, 1602–1629, https://doi.org/10.1080/00206814.2014.951978, 2014.
Li, K. Y., Shen, J. L., and Wang, X. P.: Isotopic geochronology of Mesozoic terrestrial volcanic rocks in Zhejiang, Fujian and Jiangxi China, J. Stratigr., 13, 1–13, 1989 (in Chinese with English abstract).
Li, L. M., Sun, M., Xing, G. F., Zhao, G. C., Zhou, M. F., Wong, J., and Chen, R.: Two late Mesozoic volcanic events in Fujian Province: constraints on the tectonic evolution of southeastern China, Int. Geol. Rev., 51, 216–251, 2009.
Li, S. Z., Suo, Y. H., Li, X. Y., Zhou, J., Santosh, M., Wang, P. C., Wang, G. Z., Guo, L. L., Yu, S. Y., Lan, H. Y., Dai, L. M., Zhou, Z. Z., Cao, X. Z., Zhu, J. J., Liu, B., Jiang, S. H., Wang, G., and Zhang, G. W.: Mesozoic tectono-magmatic response in the East Asian ocean-continent connection zone to subduction of the Paleo-Pacific Plate, Earth-Sci. Rev., 192, 91–137, 2019.
Li, X. H.: Cretaceous magmatism and lithospheric extension in Southeast China, J. Asian Earth Sci., 18, 293–305, 2000.
Li, X. H., Liu, X. M., Liu, Y. S., Su, L., Sun, W. D., Huang, H. Q., and Yi, K.: Accuracy of LA-ICPMS zircon U-Pb age determination: An inter-laboratory comparison, Sci. China Earth Sci., 58, 1722–1730, https://doi.org/10.1007/s11430-015-5110-x, 2015.
Li, X. H., Zhang, C. K., Li, Y. X., Wang, Y., and Liu, L.: Refined chronostratigraphy of the late Mesozoic terrestrial strata in South China and its tectono-stratigraphic evolution, Gond. Res., 66, 143–167, 2019.
Li, Z. X., and Li, X. H.: Formation of the 1300-km-wide intercontinental orogen and postorogenic magmatic province in Mesozoic South China: A flat-slab subduction model, Geology, 35, 179–182, 2007.
Liu, K., Zhang, J. J., Wilde, S. A., Zhou, J. B., Wang, M., Ge, M. H., Wang, J. M., and Ling, Y. Y.: Initial subduction of the Paleo-Pacific Oceanic plate in NE China: Constraints from whole-rock geochemistry and zircon U-Pb and Lu-Hf isotopes of the Khanka Lake granitoids, Lithos, 274–275, 254–270, 2017.
Liu, L., Xu, X. S., and Zou, H. B.: Episodic eruptions of the Late Mesozoic volcanic sequences in southeastern Zhejiang, SE China: petrogenesis and implications for the geodynamics of paleo-Pacific subduction, Lithos, 154, 166–180, 2012.
Liu, L., Xu, X. S., and Xia, Y.: Cretaceous Pacific plate movement beneath SE China: Evidence from episodic volcanism and related intrusions, Tectonophysics, 614, 170–184, 2014.
Liu, L., Xu, X. S., and Xia, Y.: Asynchronizing paleo-Pacific slab rollback beneath SE China: Insights from the episodic Late Mesozoic volcanism, Gond. Res., 37, 397–407, 2016.
Ludwig, K. R.: Squid 1.02: A User's Manual (2), Berkeley Geochron. Centre, Special Publication, 1–19, 2001.
Ma, Z. L., Li, J. H., Zhang, Y. Q., Dong, S. W., Song, C. Z., and Li, Y.: Geochronological and structural constraints on the lithostratigraphic units of the Lishui Basin, southeastern China, Geol. China, 43, 56–71, 2016 (in Chinese with English abstract).
Meng, L. F., Li, Z. X., Chen, H. L., Li, X. H., and Wang, X. C.: Geochronological and geochemical results from Mesozoic basalts in southern South China Block support the flat-slab subduction model, Lithos, 132–133, 127–140, 2012.
Qiu, Y. M., Gao, S., McNaughton, N. J., Groves, D. I., and Ling, W. L.: First evidence of N3.2 Ga continental crust in the Yangtze Craton of South China and its implications for Archean crustal evolution and Phanerozoic tectonics, Geology, 28, 11–14, 2000.
Schoene, B., Condon, D. J., Morgan, L., and McLean, N.: Precision and Accuracy in Geochronology, Elements, 9, 19–24, https://doi.org/10.2113/gselements.9.1.19, 2013.
Sha, J. G.: Plicatounio from Hekou Formation of Hekou basin, Ninghua, Fujian, with discussion on classification of Plicatounionidae, Acta Palaeont. Sin., 29, 472–489, 1990 (in Chinese with English abstract).
Shu, L. S., Zhou, X. M., Deng, P., Wang, B., Jiang, S. Y., Yu, J. H., and Zhao, X. X.: Mesozoic tectonic evolution of the Southeast China Block, New insights from basin analysis, J. Asian Earth Sci., 34, 376–391, 2009.
Shu, X., Yang, S. Y., Jiang, S. Y., and Ye, M.: Petrogenesis and geodynamic setting of Early Cretaceous felsic rocks in the Gan-Hang Belt, Southeast China: Constraints from geochronology and geochemistry of the tuffs and trachyandesitic rocks in Shengyuan volcanic Basin, Lithos, 284–285, 691–708, 2017.
Solari, L. A., Gómez-Tuena, A., Bernal, J. P., Pérez-Arvizu, O., and Tanner, M.: U-Pb zircon geochronology with an integrated LA-ICP-MS microanalytical workstation: Achievements in precision and accuracy, Geostand. Geoanal. Res., 34, 5–18, 2010.
Stepashko, A. A.: The Cretaceous Dynamics of the Pacific Plate and Stages of Magmatic Activity in Northeastern Asia, Geotectonics, 40, 225–235, 2006.
Sun, M. D., Chen, H. L., Zhang, F. Q., Wilde, S. A., Dong, C. W., and Yang, S. F.: A 100 Ma bimodal composite dyke complex in the Jiamusi Block, NE China: An indication for lithospheric extension driven by Paleo-Pacific roll-back, Lithos, 162, 317–330, 2013.
Sun, M. D., Xu, Y. G., Wilde, S. A., and Chen H. L.: Provenance of Cretaceous trench slope sediments from the Mesozoic Wandashan Orogen, NE China: Implications for determining ancient drainage systems and tectonics of the Paleo-Pacific, Tectonics, 34, 1269–1289, https://doi.org/10.1002/2015TC003870, 2015.
Sun, W. D., Ding, X., Hu, Y. H., and Li, X. H.: The golden transformation of the Cretaceous plate subduction in the west Pacific, Earth-Planet. Sci. Lett., 262, 533–542, 2007.
Taylor, B. and Hayes, D.E.: Origin and history of the South China Sea Basin: in: The Tectonic and Geologic Evolution of Southeast Asian Seas and Islands: Part 2, edited by: Hayes, D. E., Am. Geophys. Union Geophys. Monogr., 27, 23–56, 1983.
Wang, D. Z., Zhou, J. C., Qiu, J. S., and Fan, H. H.: Characteristics and petrogenesis of late Mesozoic granitic volcanic-intrusive complexes in southeastern China, Geol. J. China Uni., 6, 487–798 (in Chinese with English abstract), 2000.
Wang, G. C., Jiang, Y. H., Liu, Z., Ni, C. Y., Qing, L., Zhang, Q., and Zhu, S. Q.: Multiple origins for the Middle Jurassic to Early Cretaceous high-K calc-alkaline I-type granites in northwestern Fujian province, SE China and tectonic implications, Lithos, 246–247, 197–211, 2016.
Wang, X. L., Zhou, J. C., Qiu, J. S., Zhang, W. L., Liu, X. M., and Zhang, G. L.: LA-ICPMS U–Pb zircon geochronology of the Neoproterozoic igneous rocks from Northern Guangxi, South China: implications for tectonic evolution, Precambrian Res., 145, 111–130, 2006.
Wang, Y. J., Fan, W. M., Cawood, P. A., and Li, S. Z.: Sr–Nd–Pb isotope constraints on multiple mantle domains for Mesozoic mafic rocks beneath the South China Block hinterland, Lithos, 106, 297–308, 2008.
Wang, Y. J., Fan, W. M., Zhang, G. W., and Zhang, Y. H.: Phanerozoic tectonics of the South China Block: Key observations and controversies, Gond. Res., 23, 1273–1305, https://doi.org/10.1016/j.gr.2012.02.019, 2013.
Wu, F., Yang, J., Lo, C., Wilde, S. A., Sun, D., and Jahn, B.: The Heilongjiang Group: a Jurassic accretionary complex in the Jiamusi Massif at the western Pacific margin of northeastern China, Isl. Arc., 16, 156–172, 2007.
Wu, J. and Wu, J. H.: Shuangfengling formation in Jiangxi and its geological age, J. East China Inst. Techn., 36, 17–24, 2013 (in Chinese with English abstract).
Wu, J. H., Liu, F. Y., and Liu, Sh.: SHRIMP U-Pb Zircon Age of Late Mesozoic Trachyte in Xiajiang–Guangfeng and Sannan (Quannan, Dingnan and Longnan) – Xunwu Volcanic Belts, Geol. Rev., 57, 125–132, 2011a (in Chinese with English abstract).
Wu, J. H., Xiang, Y. X., and Liu, S.: Wuyi Group of southern Jiangxi and its geological age, J. Stratigr., 35, 200–208, 2011b (in Chinese with English abstract).
Xie, J. C., Fang, D., Xia, D., Li, Q. Z., and Sun, W. D.: Petrogenesis and tectonic implications of late Mesozoic granitoids in southern Anhui Province, southeastern China, Int. Geol. Rev., 59, 1804–1826, https://doi.org/10.1080/00206814.2017.1297964, 2017.
Yang, J. B., Zhao, Z. D., Hou, Q. Y., Niu, Y. L., Mo, X. X., Sheng, D., and Wang, L. L.: Petrogenesis of Cretaceous (133–84 Ma) intermediate dykes and host granites in southeastern China: Implications for lithospheric extension, continental crustal growth, and geodynamics of Palaeo-Pacific subduction, Lithos, 296–299, 195–211, 2018.
Yang, S. Y., Jiang, S. Y., Zhao, K. D., Jiang, Y. H., Ling, H. F., and Luo, L.: Geochronology, geochemistry and tectonic significance of two Early Cretaceous A-type granites in the Gan-Hang Belt, Southeast China, Lithos, 150, 155–170, 2012.
Yang, Y. L., Ni, P., Yan, J., Wu, C. Z., Dai, B. Z., and Yu, Y. F.: Early to late Yanshanian I-type granites in Fujian Province, SE China: Implications for the tectonic setting and Mo mineralization, J. Asian Earth Sci., 137, 194–219, 2017.
Yu, X. Q., Shu, L. S., Deng, P., Wang, B., and Zhu, F. P.: The sedimentary features of the Jurassic-Tertiary terrestrial strata in southeast China, J. Stratigr., 27, 254–263, 2003 (in Chinese with English abstract).
Zhang, B., Guo, F., Zhang, X. B., Wu, Y. M., Wang, G. Q., and Zhao, L.: Early Cretaceous subduction of Paleo-Pacific Ocean in the coastal region of SE China: Petrological and geochemical constraints from the mafic intrusions, Lithos, 334–335, 8–24, 2019.
Zhang, C., Ma, C. Q., Liao, Q. N., Zhang, J. Y., and She, Z. B.: Implications of subduction and subduction zone migration of the Paleo-Pacific Plate beneath eastern North China, based on distribution, geochronology, and geochemistry of Late Mesozoic volcanic rocks, Int. J. Earth Sci. (Geol. Rundsch.), 100, 1665–1684, 2011.
Zhang, J. H., Yang, J. H., Chen, J. Y., Wu, F. Y., and Wilde, S. A.: Genesis of late Early Cretaceous high-silica rhyolites in eastern Zhejiang Province, southeast China: A crystal mush origin with mantle input, Lithos, 296–299, 482–495, 2018.
Zhang, L. M.: The Jurassic-Cretaceous boundary in the Zhejiang-Fujian-Jiangxi region, Geol. Rev., 43, 25–31, 1997 (in Chinese with English abstract).
Zhao, G. C. and Cawood, P. A.: Tectonothermal evolution of the Mayuan assemblage in the Cathaysia Block: new evidence for Neoproterozoic collisional-related assembly of the South China craton, Am. J. Sci., 299, 309–339, 1999.
Zhao, J. L., Qiu, J. S., Liu, L., and Wang, R. Q.: The Late Cretaceous I- and A-type granite association of southeast China: Implications for the origin and evolution of post-collisional extensional magmatism, Lithos, 240–243, 16–33, https://doi.org/10.1016/j.lithos.2015.10.018, 2016.
Zheng, Y. F. and Zhang, S. B.: Formation and evolution of Precambrian continental crust in South China, Chinese Sci. Bull., 52, 1–12, 2007.
Zhou, X. M. and Li, W. X.: Origin of Late Mesozoic igneous rocks in southeastern China: implications for lithosphere subduction and underplating of mafic magmas, Tectonophysics, 326, 269–287, 2000.
Zhou, X. M., Sun, T., Shen, W. Z., Shu, L. S., and Niu, Y. L.: Petrogenesis of Mesozoic granitoids and volcanic rocks in South China: a response to tectonic evolution, Episodes, 29, 26–33, 2006.