the Creative Commons Attribution 4.0 License.
the Creative Commons Attribution 4.0 License.
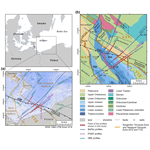
Post-Caledonian tectonic evolution of the Precambrian and Paleozoic platform boundary zone offshore Poland based on the new and vintage multi-channel reflection seismic data
Michal Malinowski
Stanisław Mazur
Sergiy Stovba
Małgorzata Ponikowska
Christian Hübscher
The structure of the post-Caledonian sedimentary cover in the transition from the Precambrian to the Paleozoic platforms in the Polish sector of the Baltic Sea is a matter of ongoing debate due to the sparsity of quality seismic data and insufficient well data. The new high-resolution BalTec seismic data acquired in 2016 contributed greatly to deciphering the regional geology of the area. Here we show an optimal seismic data-processing workflow for the selected new BalTec seismic profiles offshore Poland, as well as legacy PGI97 regional seismic data. Due to the acquisition in a shallow-water environment, the processing strategy focused on suppressing multiple reflections and guided waves through a cascaded application of modern multiple elimination approaches. We illustrate the potential of the new and re-processed data for focusing seismic interpretation on the area of the Koszalin Fault. In light of the available data, the Koszalin Fault was the main structure controlling Mesozoic subsidence and Late Cretaceous–Paleocene inversion of the eastern portion of the Mid-Polish Trough offshore Poland. The inversion changed its character from thin- to thick-skinned towards the north, away from the Polish coast. The Koszalin Fault reactivated older structural grain inherited from the time of Devonian continental rifting at the margin of Laurussia. The fault runs obliquely to the Caledonian Deformation Front, the feature that remained inactive since its formation at the Silurian–Devonian transition.
- Article
(20294 KB) - Full-text XML
-
Supplement
(2504 KB) - BibTeX
- EndNote
The transition from the East European Platform to the Paleozoic Platform of western Europe has been a matter of lively discussion for a long time (e.g., Berthelsen, 1998; Pharaoh, 1999; Thybo, 2001; Bayer et al., 2002; Mazur et al., 2016; Janik et al., 2022; Ponikowska et al., 2024, and references therein). Besides the Teisseyre–Tornquist Zone (TTZ) which is considered a boundary between the two platforms, there are additional structural elements that contribute to the complexity of the transition zone such as the Caledonian Deformation Front (CDF; Berthelsen, 1998; Katzung et al., 1993; Lassen et al., 2001; Krawczyk et al., 2002) or late Paleozoic tectonic grabens (Krzywiec et al., 2022a). These features are particularly apparent in the southern Baltic Sea where the CDF diverges away from the TTZ (Fig. 1a), and a mosaic of various geological blocks, separated by several fault zones, was formed throughout the late Paleozoic and Mesozoic (e.g., Erlström et al., 1997; Seidel et al., 2018; Ponikowska et al., 2024). These structures were produced by consecutive tectonic events including, among others, the emplacement of the Caledonian fold-and-thrust belt (e.g., Mazur et al., 2016), the late Paleozoic extension west of the TTZ (Krzywiec et al., 2022a), the Mesozoic extension (e.g., Krzywiec et al., 2003; Mazur et al., 2005), and finally the Late Cretaceous–Paleocene basin inversion (e.g., Krzywiec, 2002; Krzywiec et al., 2003, 2022b; Al Hseinat and Hübscher, 2017; Pan et al., 2022).
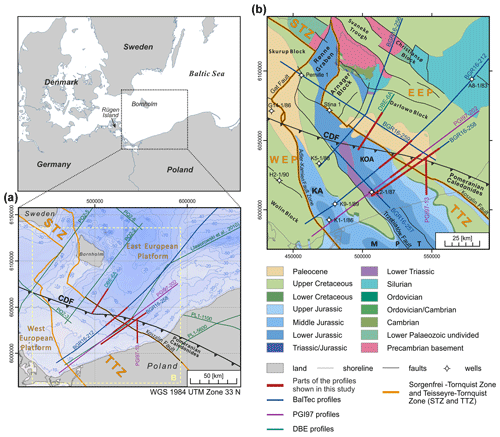
Figure 1Location of the studied seismic profiles overlaid on main structural elements of the southern Baltic Sea. (a) The bathymetry of the southern Baltic Sea and the location of seismic lines (red lines) were processed and shown in the study. Additionally, the location of the other deep-seismic profiles from previous investigations are given, namely DEKORP-PQ (PQ2-2 to PQ2-6) offshore Bornholm, Denmark (DEKORP-BASINResearch Group, 1999); a regional cross section from Jaworowski et al. (2010); and PolandSPAN™ PL1-1100 and PL1-5600 onshore Poland (Mazur et al., 2015, 2016) are shown in green. (b) Geological map of the southern Baltic Sea without post-Paleocene sediments after Kramarska et al. (1999), Schlüter et al. (1998), and Sopher et al. (2016), as well as a Pre-Quaternary map of Bornholm (Hansen and Poulsen, 1977). The position of the main faults and tectonic blocks, as well as the Teisseyre–Tornquist and Sorgenfrei–Tornquist zones, are adapted from Seidel et al. (2018). Abbreviations are as follows: CDF is for Caledonian Deformation Front; EEC is for East European Craton; WEP is for West European Platform; KA is for Kamień Anticline; KOA is for Kołobrzeg Anticline; MPT is for Mid-Polish Trough; STZ is for Sorgenfrei–Tornquist Zone; TTZ is for Teisseyre–Tornquist Zone.
Seismic imaging remains the most effective tool for resolving the complexity of superimposed structural elements in the transition zone from the Precambrian to Paleozoic platforms in the southern Baltic Sea. However, sedimentary succession in the Polish sector of the southern Baltic Sea has so far been poorly explored by low-quality industry single-channel and multi-channel seismic (MCS) reflection profiles and a few boreholes (Pokorski, 2010; Jaworowski et al., 2010). The best regional coverage (in Polish but also in German and Danish sectors) is offered by a high-resolution dataset acquired in 1996–1997 in the southern Baltic Sea (PGI97; Kramarska et al., 1999). These data mostly document the shallow Cenozoic and Mesozoic sedimentary strata (down to 0.8 s two-way time, TWT) and only in a few places reach the base of the Mesozoic (Krzywiec et al., 2003, 2002). Since the initial interpretation by Krzywiec et al. (2003), no attempts have been made to re-process and re-interpret these data. In March 2016, 3500 km of new MCS profiles were acquired on board research vessel (R/V) Maria S. Merian throughout a large area of the Baltic Sea from the Bay of Kiel to the northeast of Bornholm (Hübscher et al., 2017; Hübscher, 2018) (cruise MSM52; also known as BalTec). Thanks to the unique acquisition parameters, the BalTec MCS data image the tectonic elements from the seafloor down to the Paleozoic basement. With its large geographic coverage and resolution, these seismic data provide better capabilities for visualizing geological features and mapping structural formations than previous datasets (see examples of new interpretation in, e.g.,Ahlrichs et al., 2020, 2022; Janik et al., 2022; Krzywiec et al., 2022b; Nguyen et al., 2023).
The aim of this contribution is twofold. First, we illustrate the efficiency of processing work for the newly acquired BalTec seismic data. Furthermore, we investigate if modern processing tools can improve the quality of the legacy PGI97 data and to what extent these data can supplement the interpretations derived from the BalTec profiles. Finally, with the newly processed and re-processed data, we perform seismic interpretation to put some constraints on the structural pattern of sedimentary successions in the transition zone from the Precambrian to Paleozoic platforms. The central part of the interpretation is the tectonic evolution of the Koszalin Fault and its relation to the CDF offshore Poland.
The southern Baltic Sea area is located in the transition zone between the Fennoscandian Shield as part of the East European Craton (EEC) and the West European Platform (WEP). This area is characterized by a mosaic of various geological blocks separated by several faults and fault zones formed throughout the Phanerozoic (Liboriussen et al., 1987; Vejbaek et al., 1994; Berthelsen, 1998; Pharaoh, 1999; Thybo, 1999; van Wees et al., 2000). The most prominent tectonic features are the NW–SE-trending Sorgenfrei–Tornquist and Teisseyre–Tornquist Zones (STZ and TTZ) crossing the southern Baltic Sea to the north and south of Bornholm, respectively (Fig. 1a; Berthelsen, 1998; Pharaoh, 1999). These zones are characterized by major, often deeply rooted faults that governed the subsidence and uplift of major crustal blocks during several tectonic phases in the Paleozoic, Mesozoic, and, locally, Cenozoic (Vejbaek et al., 1994; Dadlez et al., 1995; Krzywiec, 2002; Krzywiec et al., 2003).
At the southwestern margin of the EEC, Proterozoic granites and gneisses are overlain by Cambro–Ordovician sediments of the Baltica passive margin as penetrated by offshore wells (e.g., Franke et al., 1994; Beier and Katzung, 1999). The closure of the Tornquist Sea and the subsequent collision of Baltica with Avalonia during the Late Ordovician led to the formation of the Caledonian fold-and-thrust belt (Katzung et al., 1993; Dallmeyer et al., 1999; Katzung, 2001; Torsvik and Rehnström, 2003). In this context, the Cambro–Ordovician successions of the Baltica margin were overthrust by deformed marine Ordovician sediments (Katzung et al., 1993). The formation of an accretionary wedge is indicated by seismic surveys offshore Rügen island (Babel Working Group, 1993; Thomas et al., 1993; Piske et al., 1994; Schlüter et al., 1997; DEKORP-BASINResearch Group, 1999). In addition, evidence of deformed Ordovician sediments of marine origin was found in several deep wells on Rügen island (e.g., Dallmeyer et al., 1999; Katzung, 2001). The northernmost extension of the Caledonian fold-and-thrust belt is marked by the CDF, which can be traced from Lolland and Møn (Denmark) to the north of Rügen island and farther towards northwestern Poland (Fig. 1a; Liboriussen et al., 1987; Erlström et al., 1997). The CDF onshore NW Poland is marked out by a thin-skinned fold-and-thrust belt involving Ordovician and Silurian mudrock succession of the Pomeranian Caledonides (Mazur et al., 2016). The oldest sediments are late Llanvirn and Caradoc in age, and they are accompanied by fragments of the entire Silurian profile up to the Pridoli (Podhalańska and Modliński, 2010). This is in contrast to the Rügen area, where only thick (∼1500–2000 m) Ordovician sediments have been drilled (e.g., Katzung et al., 1993), including Tremadoc–Llanvirn sandstones, shales, and greywackes that experienced anchizonal metamorphic conditions (Dallmeyer et al., 1999). Therefore, the Pomeranian Caledonides with their potential offshore continuation are considered part of the Caledonian foreland basin that was deformed and included in the orogenic wedge during the late stages of Caledonian shortening (Mazur et al., 2016). Consequently, the CDF separates the undeformed succession of lower Paleozoic sediments in the northeast, referred to as the Baltic Basin, from the Caledonian fold-and-thrust belt in the southwest (Fig. 1a). The latter forms the basement of the WEP that is unconformably covered by Devonian–Carboniferous and Permian–Mesozoic successions.
The NW–SE-trending Koszalin Fault is regarded as the offshore prolongation of the eastern boundary of the Koszalin–Chojnice Structural Zone (KCSZ). Geological interpretation of the LT-7 deep-refraction profile (Guterch et al., 1994) shows the Koszalin Fault as the distal antithetic break-off in the hanging wall (Berthelsen, 1998); other antithetic faults rotate the downfaulted basement and pre-Permian cover southwest of the Koszalin Fault (Antonowicz et al., 1994). Offshore Poland, the Koszalin Fault delimits the boundary between the Darłowo and Kołobrzeg tectonic blocks, and it is associated with deformations in the sub-Permian and Mesozoic strata. The strongly localized subsidence occurred mostly in the Triassic and Jurassic periods, presumably as a result of local strike-slip displacements, transtension, and the creation of small pull-apart basins (Pietsch and Krzywiec, 1996; Krzywiec, 2002). Later, due to NE–SW compression, this entire tectonic system was inverted during the Late Cretaceous and Paleocene time (Antonowicz et al., 1994). The extensional fault system reactivated reversely in the pre-Zechstein basement is accompanied by asymmetric fault propagation folds generated within the Mesozoic infill (Schlüter et al., 1997; Dadlez et al., 1995; Kramarska et al., 1999; Krzywiec, 2002).
The BalTec 2D MCS data were acquired in March 2016 on board R/V Maria S. Merian by the Federal Institute for Geosciences and Natural Resources Germany (BGR) (for details, see Hübscher et al., 2017). The main acquisition parameters and an example of a raw-shot gather are presented in Table 1 and Fig. 2a, respectively. Around 850 km of the BalTec data were recorded in the Polish Exclusive Economic Zone and territorial waters. The location of the selected BalTec profiles is shown in Fig. 1b.
Table 1Acquisition parameters for the two seismic surveys: BalTec and PGI97. DGPS stands for Differential Global Positioning System.
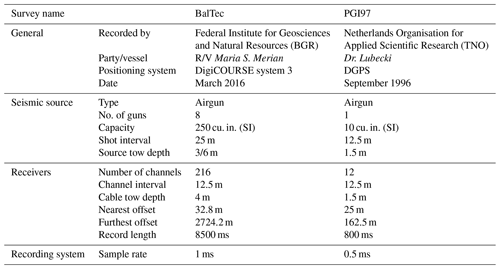
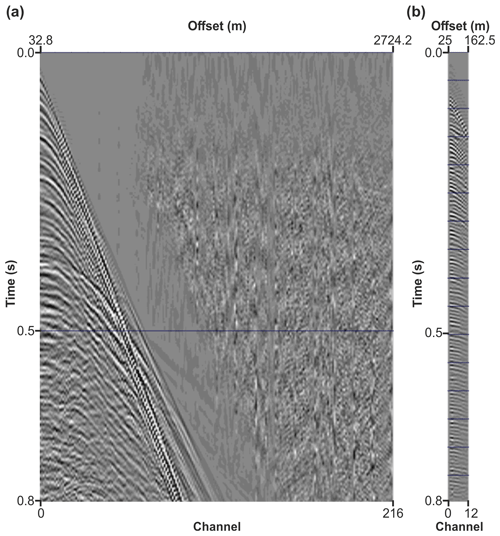
Figure 2Raw-shot gathers from the two different surveys BalTec (a) and PGI97 (b). Note the large difference in terms of the number of channels (and offsets) between the two datasets.
The PGI97 2D MCS data were acquired in 1996–1997 as part of a collaboration of the Polish Geological Institute (PGI) and the Netherlands Organisation for Applied Scientific Research (TNO) (Kramarska et al., 1999; Krzywiec et al., 2003). This project aimed to image the geology of the upper 400 m beneath the sea bottom to prepare a new geological map of the Baltic Sea (Kramarska et al., 1999). The dataset consists of several profiles extending to German, Danish, and Swedish sectors, with a total length of 4000 km. The main acquisition parameters and an example of a raw-shot gather are shown in Table 1 and Fig. 2b, respectively. Two profiles were selected to complement the BalTec data for interpretation purposes in this study. Available pre-stack data (raw-shot gathers) transcribed from original tapes by PGI make the re-processing possible.
BalTec and PGI97 data were complemented by the DBE (Danish Bornholm Enclave) dataset acquired offshore Bornholm in 1982 by Western Geophysical. Geological Survey of Denmark and Greenland (GEUS) provided us with the selected profiles (21 profiles in the Danish territorial waters). Only post-stack time-migrated versions were available.
In this study, in order to image the Koszalin Fault, CDF, and the sedimentary cover overlying TTZ, five profiles were selected from three different datasets (see Fig. 1 for locations), namely two profiles from the BalTec data (BGR16-258 and BGR16-212), two profiles from the PGI97 data (PGI97-13 and PGI97-202), and one profile from the DBE data (DBE-6A). The selected sections run from the vicinity of the Polish coast toward the Amager tectonic block in the Danish waters. Most of the seismic sections are oriented from the northeast to the southwest and perpendicular to the Koszalin Fault. Unfortunately, well data information is very limited for this study. Only the SW end of profile BGR16-258 is calibrated by deep-well L2-1/87 (Fig. 1b). The borehole information is limited offshore Pomerania because this area is considered less favorable for hydrocarbon exploration (Karnkowski et al., 2010). However, our seismic interpretation relies on publicly available data from all offshore wells shown in Fig. 1: G14-1/86 (Seidel et al., 2018), Pernille-1, Stina-1 (Vejbaek et al., 1994; Graversen, 2004), K9-1/86, K1-1/86, L2-1/87, and A8-1/83 (Rempel, 1992; Schlüter et al., 1997; Krzywiec, 2002).
Here we provide a basic description of the processing workflow applied to the new BalTec data, as well as the re-processing of the PGI97 data. More details are provided in the accompanying Supplement, including Figs. S1 to S5.
4.1 Processing of the new BalTec data
Multiple reflections are the major problem faced when processing seismic data acquired in the Baltic Sea due to the relatively shallow-water depth (average water depth around 50 m), and the new BalTec survey is not an exception. Therefore, removing multiple reflections (hereinafter referred to as demultiple) was central throughout the entire processing flow for these data. Based on analysis of the properties of multiples present in seismic data, the demultiple workflow consisted of (i) a surface-related multiple elimination (SRME) method (Verschuur, 2013), (ii) water-bottom frequency–wavenumber (F–K) filtering, (iii) predictive deconvolution in the τ-p domain, and (iv) a high-resolution parabolic Radon demultiple (Harlan, 1995). The order of applying these methods is shown in Table 2 and discussed in more detail in the Supplement. The effectiveness of our approach is illustrated in Figs. S1 and S2. The cascaded demultiple approach in the BalTec processing workflow improved the velocity analysis and helped to produce the final pre-stack time-migrated (PSTM) stack sections optimal for subsequent seismic interpretation. Even though the structures in the area are only moderately dipping, we evaluated the PSTM approach as superior to the post-stack time migration used during on-board processing (Fig. 3). More details about the velocity analysis, together with the examples of the velocity models (Figs. S4 and S5) and the details on PSTM, are provided in the Supplement.
Table 2Processing workflow applied to BalTec data. SEG-D stands for Society of Exploration Geophysicists Digital Type Format, F–X stands for frequency–distance, and AGC stands for automatic gain control.
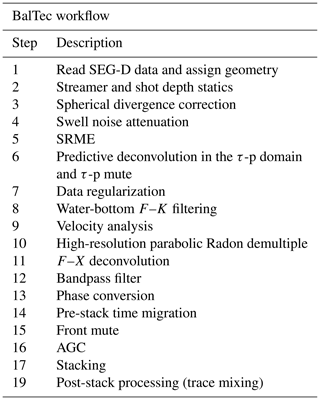
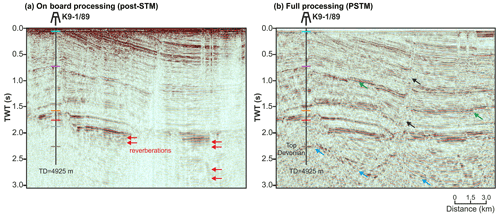
Figure 3Comparison of BalTec on-board processing (Hübscher et al., 2017) (a) and full processing (b) along part of line BGR16-212. Post-stack time migration and pre-stack time migration were applied in the on-board processing and the full processing, respectively. Particular image enhancements are highlighted by arrows. Note that the stack in panel (b) has an AGC applied to enhance structural interpretation.
4.2 Re-processing of the vintage PGI97 dataset
The PGI97 data were processed at TNO shortly after the survey (see the original processing flow in Table 3). The original processing was limited in demultiple approaches, especially before stacking, due to the limited demultiple techniques available at that time and the limitations of the data (short offsets). An example of stacked sections created using the original workflow is shown in Fig. 4a. Reverberations from the primary events are dominant across the seismic section. Additionally, the existence of high-frequency noise can be easily seen in the section, which can lead to the misinterpretation of the main geological features. Previously, sections were stacked using a simple-velocity model, and no migration process was applied. Re-processing (see the workflow in Table 3) addressed all the above issues.
Table 3Re-processing workflow applied to PGI97 data and the original processing workflow of PGI97 data. SEG-Y stands for Society of Exploration Geophysicists Exchange Tape Format, and NMO stands for normal move-out.
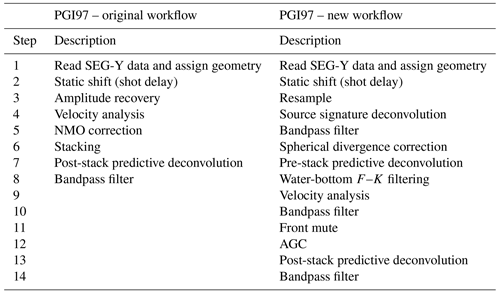
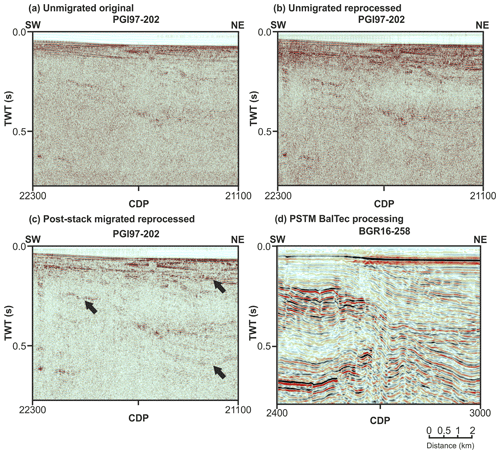
Figure 4Comparison of part of the final stack sections of the vintage PGI97-202 profile: unmigrated original (a), unmigrated re-processed (b), and migrated re-processed (c), together with the parallel new BGR16-258 profile (d) (see Fig. 1 for location). Black arrows mark the noticeable enhancement in the quality of re-processed section.
Since the PGI97 data have a very short maximum offset (150 m) (Fig. 2b), the move-out-based (Radon demultiple) or model-based demultiple approaches (SRME) were not applied to these data. Only the predictive deconvolution approach was attempted before and after stacking. It efficiently attenuates severe reverberations and water-bottom multiples (Fig. S3) and helps in velocity analysis. Although the velocity analysis was limited by the available offset, the new velocity models (see example in Fig. S6) were considered robust enough and conforming to the geological structure to produce improved stack sections. The data were then migrated by a post-stack, finite, and different time migration routine (Claerbout and Doherty, 1972) using the new smoothed velocity model (Fig. 4c).
Compared with the on-board processing results (Hübscher et al., 2017) (Fig. 3a), the new processing of BalTec data (Fig. 3b) significantly enhanced reflection events (green arrows in Fig. 3b), fault planes (black arrows in Fig. 3b), and, more importantly, deep-reflection events (e.g., the top Devonian represented by blue arrows in Fig. 3b). Short-path multiples (red arrows in Fig. 3a) were also suppressed in the fully processed version. It can be attributed to the interplay of multiple elimination, improved velocity analysis, and pre-stack time migration (as opposed to the post-stack migration used during on-board processing).
The re-processing of the PGI97 data brings significant improvement in the signal-to-noise ratio, especially in the final, migrated, and stacked image (Fig. 4c). Since the overall continuity of reflection events is improved, fault displacements are much easier to interpret. Dipping events are imaged at their true locations after migration. However, despite a lot of effort in the re-processing workflow to suppress multiples, the remnants of multiples still exist in the data. The parallel profile BGR16-258 (Fig. 4d) provides a much better image of the sediments in the Koszalin Fault area compared to the profile PGI97-202.
Differences in terms of both the vertical and horizontal resolution between the BalTec and PGI97 data are related to the acquisition parameters and configurations adjusted to the purpose of the two datasets. Even though both data have a nominal common depth point (CDP) spacing of 6.25 m (forced to 12.5 m for BalTec), the frequency content is drastically different, with 100–400 Hz for PGI97 and 8–80 Hz retained in the current processing of BalTec data.
The final PSTM sections of BalTec data demonstrate some distinct reflection patterns for each of the units (Fig. 5). In the EEC, the Precambrian crystalline basement is represented by a chaotic pattern with low- to medium-amplitude events. The basement is overlaid by thin Cambro–Ordovician formation (∼0.2 s TWT) represented by semi- to highly continuous parallel reflections with medium to very high amplitude. On top of the Ordo–Cambrian formation, a thick Silurian succession (from 1.0 to 1.5 s TWT) is characterized by chaotic and continuous parallel reflections with low to high amplitude. In the WEP, the boreholes penetrate solely down to Devonian. Therefore, in this study, all sedimentary successions below are called pre-Carboniferous formation. The pre-Carboniferous formation is a mixture of chaotic and discontinuous reflection patterns with low- to medium-amplitude events, while the Carboniferous formation (thickness ∼0.5 s TWT) is represented by an inclined parallel reflection pattern. The Permian formation is composed of very high-amplitude events. Locally very thin Rotliegend deposits are overlaid by medium- to high-amplitude Zechstein. Reflection patterns of the thick Mesozoic deposits (∼1 to 2 s TWT) are relatively consistent by semi- to highly continuous parallel events with low to high amplitudes.
The seismic interpretation is presented for selected five profiles (BGR16-212, BGR16-258, DBE-6A, PGI97-202, and PGI97-13; Figs. 6–10). As mentioned before, there is very sparse well control in the study area. For this reason, the interpretation of these five profiles was carried out together with all other offshore profiles shown in Fig. 1b, and all seismic profiles were tied to available well data in the study area. The seismic fabrics, reflecting the structural features of the sedimentary cover at the locations of three of these wells, are shown in Fig. 5. In the seismic sections, the horizons are interpreted as the base of each formation. It is worth noting that previous research was taken into account in the research process in the southwestern part of the Baltic Sea close to the Koszalin Fault (e.g., Vejbaek et al., 1994; Krzywiec et al., 2003; Graversen, 2004; Pokorski, 2010; Jaworowski et al., 2010). For consistency, the interpretation was performed using the migrated time sections; however, depth-converted sections were also available (see an example for line BGR16-212 in Fig. S7; Ponikowska et al., 2024).
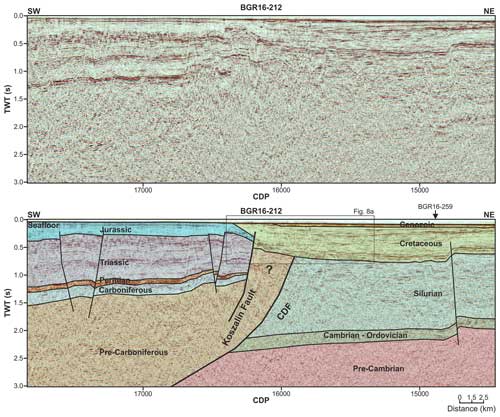
Figure 6Uninterpreted and interpreted part of BalTec line BGR16-212 (see Fig. 1 for the location). The question mark represents no possibility for predicting the origin of the reflectors.
6.1 Profile BGR16-212
The section of the BGR16-212 profile used in this study crosses the CDF and the Koszalin Fault (Fig. 1). The latter appears as a major inversion feature causing uplift of Jurassic strata onto the seafloor surface SW of the fault (Fig. 6). The uplifted Jurassic forms a core of the major inversion-related fold, the Kołobrzeg Anticline (Fig. 1b). The anticline represents the offshore continuation of the Mid-Polish Anticlinorium that splits into the Kołobrzeg and Kamień anticlines NW of the Polish coast (Fig. 1b). The lack of Cretaceous strata suggests deep syn-inversion erosion. The amount of uplift cannot be estimated since the original thickness of the Jurassic and Cretaceous remains unknown. However, the minimum amount of uplift is constrained to 0.7 s TWT (∼1 km) by the top of the pre-Carboniferous and top of the Jurassic. The presence of extensive Triassic and Jurassic strata, as well as thin Zechstein and Carboniferous layers, on the hanging wall of the reversed Koszalin Fault indicates that the latter was originally formed as a normal dip-slip fault (Fig. 6). The minimum downthrown of the hanging wall can be estimated to 1.3 s TWT (∼1.7 km), but this amount is only a minimum measure as a missing part of the Jurassic and entire Cretaceous is not included. Furthermore, the amount of pre-Zechstein erosion remains unconstrained.
The footwall of the Koszalin Fault is onlapped by a Late Cretaceous syn-inversion marginal trough (Fig. 8a). Seismic events within the footwall show that the thickness change occurs away from the Koszalin Fault, indicating syn-kinematic deposition during Late Cretaceous inversion (Fig. 8a). The trough fringes the uplifted core of the Kołobrzeg Anticline, attaining a thickness of 0.7 s TWT, i.e., ∼1 km. The rim of the Late Cretaceous trough onlaps the Koszalin Fault and the margin of the Kołobrzeg Anticline (Fig. 6). Cretaceous sediments in the footwall are deposited directly on the Silurian substratum. This implies that the footwall was uplifted and eroded throughout the late Paleozoic and Mesozoic until the late Cretaceous. The exact timing of the uplift and erosion cannot be determined from the profile, and this issue is addressed in Sect. 7.
The CDF coincides with the overthrust of the thrust-folded lower Paleozoic on the mostly undeformed lower Paleozoic strata of the Baltic Basin. The thrust has not been reactivated during the Late Cretaceous inversion. The amplitude of the overthrust, as well as the timing of deformation, cannot be inferred from seismic data. Nevertheless, the stratigraphic record (Podhalańska and Modliński, 2010) points to the Silurian–Devonian transition as the time of folding and thrusting. The Caledonian thrust is of a thin-skinned character (Mazur et al., 2016), which is a feature that is not entirely clear from profile BGR16-212. In the profile, the CDF coincides with the basement slope, forming a frontal ramp of the thrust system (Fig. 6). SW of the CDF, the top of the basement is located below the extent of the seismic data.
6.2 Profile BGR16-258
There are several similarities between profiles BGR16-258 and BGR16-212 (Figs. 6 and 7). The Koszalin Fault again appears as a major inversion feature uplifting Jurassic strata to the seafloor surface SW of the fault. At the extreme SW of the profile, the Triassic is exposed at the sea bottom (Fig. 7). The uplifted Triassic and Jurassic form a core of the major inversion-related Kołobrzeg Anticline (Fig. 1b). The lack of Cretaceous and, in the far SW, also Jurassic strata suggests deep syn-inversion erosion. Only a minimum amount of uplift can be estimated since the original thickness of the Jurassic and Cretaceous remains unknown. Minimum uplift is constrained to 1 s TWT (∼1.3 km) by the top of pre-Carboniferous and top of Jurassic. The presence of extensive Triassic and Jurassic strata, as well as Zechstein, Carboniferous, and Devonian layers on the hanging wall, indicates that the Koszalin Fault was originally formed as a normal dip-slip fault (Fig. 7). The minimum downthrown of the hanging wall can be estimated to 1.3 s TWT (∼1.7 km), but this is a minimum amount as missing Jurassic and Cretaceous is not considered. Moreover, two more faults, similar to the Koszalin Fault, occur farther SW. They cause another downthrow of the top of Silurian by 0.5–1 s TWT. The more southwestern of these two faults is a growth fault indicating Devonian syn-sedimentary extensional tectonics.
A Late Cretaceous syn-inversion marginal trough occupies the footwall of the Koszalin Fault (Fig. 7). The trough developed next to the uplifted Kołobrzeg Anticline, attaining a maximum thickness of 0.8 s TWT, i.e., ∼1.1 km. The set of growth layers in the Late Cretaceous trough is consistent with syn-inversion sedimentation (Fig. 8b). The margin of the trough onlaps the Koszalin Fault and the adjacent part of the Kołobrzeg Anticline (Fig. 7), suggesting a continuation of erosion and subsidence sometime after the cessation of inversion. Cretaceous sediments cover a regional top Silurian unconformity, proving the deep pre-inversion erosion of the present-day footwall of the Koszalin Fault.
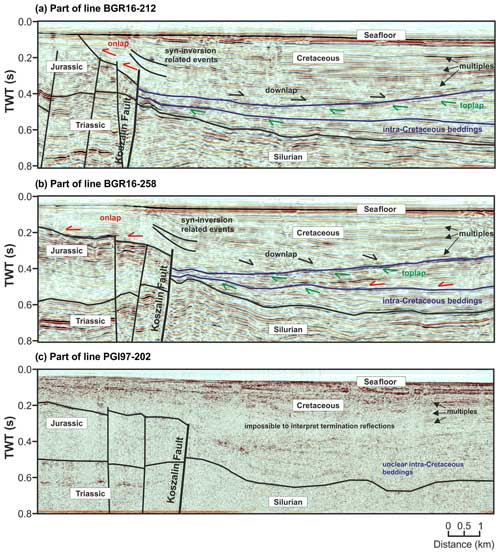
Figure 8Enlarged part of seismic section lines BGR16-212 (a) and BGR16-258 (b) demonstrates the geometry of the reflections within the Cretaceous formation that is related to the inversion movements of the Koszalin Fault. Similar geometry of reflections cannot be interpreted in the legacy PGI97-202 line (c) due to much lower-quality data.
The CDF coincides with the overthrust of the deformed lower Paleozoic of the Pomeranian Caledonides on the tectonically undisturbed lower Paleozoic of the Baltic Basin. As in line BGR16-212, there are no indications of the inversion-related reactivation of the Caledonian thrust. The latter is imaged as a relatively sharp boundary separating the orogenic wedge from the undeformed foreland. A nearby analogue of such a structure is the Carpathian thrust front in SE Poland, where a relatively sharp frontal thrust separates deformed sediments of the Carpathian foredeep from their undeformed equivalents (Krzywiec, 2001; Gągała et al., 2012). The Caledonian thrust branches off from the top of the basement (Fig. 7), the slope of which forms a frontal ramp for the Caledonian fold-and-thrust belt (Mazur et al., 2016). The top of the basement slopes southwestward below the extent of the seismic data.
6.3 Profile DBE-6A
This is the northwesternmost profile out of the five presented in this paper (Fig. 1b). In the DBE-6A line, the CDF is not imaged since it is located farther west beyond the termination of the seismic profile. This is the case since the CDF diverges away from the Koszalin Fault and turns toward the WNW (Fig. 1b). The Koszalin Fault is imaged again as an important inversion structure (Fig. 9). Although in a map-scale picture the fault still represents the NE limit of the Kołobrzeg Anticline, the seismic profile reveals a lower-order fault propagation fold within the hanging wall of the Koszalin Fault (Fig. 9). The top of the basement is also involved in folding that proves a thick-skinned character of deformation. The top of the basement is only slightly displaced, and there is virtually no displacement for the top of the Silurian. However, higher up, the Devonian to Jurassic strata are juxtaposed across the fault with the Upper Cretaceous fill of the marginal trough. It means that the original normal down-dip displacement of the order of 0.7 s TWT (∼0.9 km) was entirely reversed during the inversion phase. A footwall of the Koszalin Fault is onlapped by the Late Cretaceous marginal trough that is circa 0.7 s TWT thick (∼0.9 km).
Although profile DBE-6A lacks evidence for the end of Silurian and Caledonian deformation, it still documents the late Paleozoic–Mesozoic subsidence in the hanging wall of the Koszalin Fault. This is demonstrated by the presence of the Carboniferous, Zechstein, Triassic, and Jurassic layers. This structural geometry indicates that the Koszalin Fault was originally formed in the late Paleozoic as a normal dip-slip feature. Furthermore, the seismic profile indicates that the structural domain characterized by late Paleozoic or Mesozoic pre-Late Cretaceous uplift and erosion is limited from the west by the Koszalin Fault, and the CDF did not contribute to the development of the top Silurian unconformity in the area farther east.
An interesting feature represents another fault imaged near the NE termination of the profile (Fig. 9). The fault cuts through the Darłowo Block (Fig. 1b) from the top of the basement upward into the Late Cretaceous trough. This is clearly an inversion-related structure since it disturbs most of the Cretaceous succession (Fig. 9). It also causes localized uplift of the top of the Cretaceous. The unnamed fault in question is a thick-skinned feature displacing the top of the basement by 0.25 s TWT (∼0.7 km). Furthermore, the side branches jointly form a positive flower structure indicating a transpressional component during the Late Cretaceous inversion.
6.4 Profile PGI97-13 and PGI97-202
Profile PGI97-202 is parallel to the BGR16-258 line running close to the north of it. In contrast, the PGI97-13 profile adjoins the BGR16-258 line at a high angle and is also oblique to the Koszalin Fault (Fig. 1b). Both PGI97 lines image the Koszalin Fault quite schematically (Fig. 10). The fault appears as an inversion structure with a reverse displacement of the order of 0.2 s TWT. Both profiles also document the previous dip-slip kinematics of the Koszalin Fault revealed by the increased thickness of the Jurassic strata in the hanging wall (Fig. 10). As in the previously described profiles, the footwall of the Koszalin Fault is onlapped by the Late Cretaceous syn-inversion marginal trough. The PGI97 profiles image its thickness in the range of 0.7 s TWT. Similar to the remaining seismic lines presented, the marginal trough onlaps the Koszalin Fault (Fig. 10), providing evidence for erosion and subsidence continuing for some time after the termination of inversion. A characteristic feature, especially of profile PGI97-202, is the roughness of the top of the Cretaceous horizon (Fig. 10), suggesting final post-inversion uplift and erosion before the commencement of Cenozoic sedimentation.
The foundation for this study was the development of an optimal seismic data-processing workflow for the selected BalTec seismic profiles offshore Poland. Due to the acquisition in a shallow-water environment, our processing strategy focused on suppressing multiple reflections and guided waves through a cascaded application of SRME, τ-p deconvolution, water-bottom F–K filtering, and parabolic Radon multiple elimination. The processing workflow outlined here (with the details provided in the Supplement) could be easily adapted to other multichannel seismic data acquired in the Baltic Sea (or shallow water in general). To expand the coverage of the seismic database, we also investigated improving the quality of the legacy PGI97 seismic data using modern processing techniques. While the re-processed profiles did not contribute significantly to the interpretation presented in our study (mostly because of the original acquisition limitations), post-stack migrated results show significant improvement in overall data quality compared to images from the original processing presented in Krzywiec et al. (2003). On the one hand, it confirms the value of revisiting legacy data; on the other hand, the new BalTec data are strikingly better at imaging the geology of the study area (Fig. 4).
Two BGR16 profiles show that the CDF is a sharp thrust contact juxtaposing the Pomeranian Caledonides and the Caledonian foreland basin (Baltic Basin). This thrust was never reactivated during the subsequent geological history. The profiles do not contradict the interpretation postulating the thin-skinned character of the Caledonian fold-and-thrust belt (Mazur et al., 2016), but they do not provide further evidence to confirm this hypothesis. The Caledonian frontal thrust branches off from the basement slope that may represent a frontal ramp during the end Silurian shortening. However, this cannot be verified because the top of the Precambrian basement is below the range of seismic imaging (Figs. 6 and 7) on the hanging wall of the frontal overthrust.
The Koszalin Fault was originally formed due to Devonian extensional tectonics. The BGR16-258 profile shows that the fault was only the northeasternmost one among a number of extensional features downthrowing the top of Silurian toward the SW (Fig. 7). However, the Koszalin Fault is a structure that marks out the NE limit of this extensional event. Since the late Paleozoic tectonic grabens are described along-strike onshore Poland and in some other localities of the southern Baltic region (Krzywiec et al., 2022a, and references therein), the Koszalin Fault may belong to a family of structures resulting from a wide-range tectonic event. This could have been early Carboniferous rifting affecting the passive margin of Laurussia (Smit et al., 2018), a Middle Devonian continental rifting event (Ponikowska et al., 2024), or a combination of both. Close to the Polish coast (Figs. 6 and 7) and onshore Poland (Mazur et al., 2016), the Koszalin Fault is located SW of the CDF. Therefore, a narrow zone of the Caledonian basement (Chojnice Structural Zone) is accessible by wells, whereas farther SW, the top Silurian is downthrown to depths below the interval penetrated by boreholes (Fig. 11a). However, farther north, the Koszalin Fault crosses the CDF and cuts through the Precambrian Platform (Fig. 9).
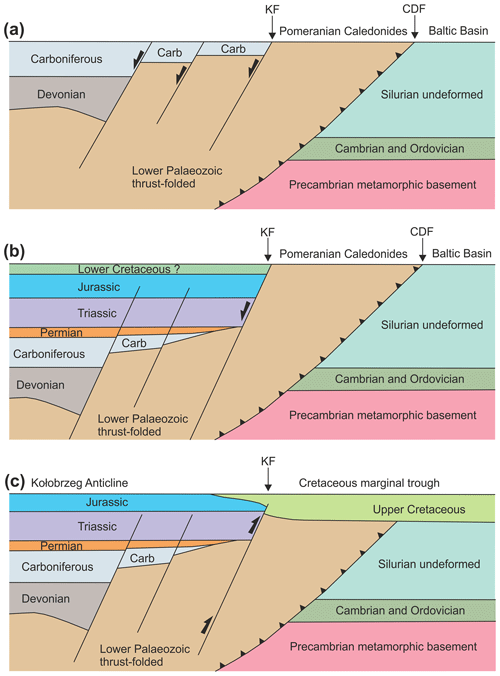
Figure 11Conceptual diagrams showing tectonic evolution of the transition zone between the Precambrian and Paleozoic Platforms offshore Poland for early Carboniferous (a), middle Cretaceous (b), and post-Paleocene (c). (a) Deposition of late Paleozoic strata west of the Pomeranian Caledonides resulted from normal dip-slip faulting initiated in the Middle Devonian and lasting until the early Carboniferous. (b) Permian–Mesozoic extension was focused on the Koszalin Fault after a period of late early to late Carboniferous uplift. (c) Late Cretaceous–Paleocene inversion formed the Kołobrzeg Anticline and Upper Cretaceous marginal trough.
A recent apatite and zircon low-temperature thermochronology study postulates an important early Carboniferous uplift in the area of the Baltic Basin NE of the CDF and Koszalin Fault (Botor et al., 2021). It remains uncertain from the seismic profiles presented whether this uplift was localized along the pre-existing Devonian faults or had a character of long-wavelength doming. The latter possibility seems more likely when observing the gradual thinning out of the Carboniferous layer toward the NE (Figs. 6 and 7). The domal-style uplift must have persisted until the end of the Permian that is shown by the eastward tapering of the Zechstein layer (Figs. 6 and 7). Therefore, the top Silurian unconformity in the area of the Baltic Basin, earlier considered a Variscan unconformity, may represent an early Carboniferous uplift event. Furthermore, the original extent of Devonian and Carboniferous sediments towards the east could be different than today.
The Koszalin Fault was certainly reactivated as a normal dip-slip feature in the Triassic (Fig. 11b). More than 1 km of Triassic sediments and several hundred meters of Jurassic were deposited during continuing Mesozoic subsidence (Figs. 6 and 7). The thickness of the later eroded Cretaceous sediments remains unconstrained. In the Mesozoic, the Koszalin Fault focused nearly all subsidence, while other faults were almost inactive (Fig. 6).
During the late Cretaceous–Paleocene basin inversion, the Koszalin Fault was reactivated as a major reverse fault (Fig. 11c). The fault limited the eastern limb of the Kołobrzeg anticline and facilitated its uplift exceeding 1 km (Fig. 1). After the activity ceased, the Koszalin Fault was covered by the marginal part of a syn-tectonic Cretaceous trough, the subsidence of which lasted longer than the inversion. The onlap of Upper Cretaceous sediments may have been related to the bending of the anticline crest.
We attempted a new seismic interpretation of the sedimentary successions overlying the crystalline basement in the transition zone from the Precambrian to Paleozoic platforms offshore Poland using the new (BalTec), as well as vintage (DBE), and re-processed legacy reflection seismic data (PGI97). Both processing and re-processing efforts were developed in-house with a particular emphasis on multiple reflection elimination. Even though the interpretation was hampered by the lack of sufficient well data control, we managed to provide some constraints on the tectonic evolution of the Koszalin Fault and its relation to the CDF.
The paper discusses geological features in the transition zone between the East European Precambrian Platform and the West European Paleozoic Platform, focusing on the CDF and the Koszalin Fault. It highlights the CDF as a thrust contact between the Pomeranian Caledonides and the Caledonian foreland basin, suggesting it remained inactive throughout subsequent geological history. The Koszalin Fault originated from Devonian extensional tectonics and may represent a broader tectonic event related to late Paleozoic continental rifting and post-rift thermal subsidence. After a period of overall uplift in the Carboniferous and early Permian, the fault experienced reactivation during the Triassic as a normal dip-slip feature. Mesozoic subsidence in the area was primarily focused along the Koszalin Fault, while other faults remained less active. During the Late Cretaceous–Paleocene, the Koszalin Fault became a major reverse fault influencing the basin inversion, growth of inversion-related anticline, and formation of the Late Cretaceous syn-inversion marginal through.
BalTec (MSM52) seismic data processed in this study are available upon request. PGI97 data are owned by the Polish State Treasury and are available via request from the Polish Ministry of Environment. Danish data are available on request from GEUS.
The supplement related to this article is available online at: https://doi.org/10.5194/se-15-1029-2024-supplement.
QN: conceptualization, methodology, data curation, writing (original draft preparation), software, and visualization. MM: supervision, project administration, and writing (review and editing). SM and SeS: data curation and writing (original draft preparation). MP: visualization and data curation. CH: project administration and data provider.
At least one of the (co-)authors is a member of the editorial board of Solid Earth. The peer-review process was guided by an independent editor, and the authors also have no other competing interests to declare.
Publisher's note: Copernicus Publications remains neutral with regard to jurisdictional claims made in the text, published maps, institutional affiliations, or any other geographical representation in this paper. While Copernicus Publications makes every effort to include appropriate place names, the final responsibility lies with the authors.
This study was funded by the Polish National Science Centre (grant no. UMO-2017/27/B/ST10/02316). Sergiy Stovba thanks the Polish National Science Centre for the financial support (grant no. UMO-2022/01/3/ST10/00030). Cruise MSM52 has been funded by the German Science Foundation DFG and the Federal Ministry of Education and Research (BMBF). We thank the Federal Institute for Geosciences and Natural Resources (BGR) for their support during seismic data acquisition and for sharing the data. DBE data were provided thanks to the agreement with the Geological Survey of Denmark and Greenland (GEUS).
We would like to thank IHS Markit Ltd and Petrosys Pty Ltd for the donation of academic licenses for the Kingdom suite and GLOBEClaritas software packages, respectively.
We thank the two anonymous reviewers for their helpful comments that improved the paper.
This study has been funded by the Polish National Science Centre (grant no. UMO-2017/27/B/ST10/02316). Sergiy Stovba thanks the Polish National Science Centre for the financial support (grant no. UMO‐2022/01/3/ST10/00030). Cruise MSM52 has been funded by the German Science Foundation DFG and the Federal Ministry of Education and Research (BMBF). We thank the Federal Institute for Geosciences and Natural Resources (BGR) for their support during seismic data acquisition and sharing of the data. DBE data were provided thanks to the agreement with the Geological Survey of Denmark and Greenland (GEUS).
This paper was edited by Irene Bianchi and reviewed by two anonymous referees.
Ahlrichs, N., Hübscher, C., Noack, V., Schnabel, M., Damm, V., and Krawczyk, C. M.: Structural Evolution at the Northeast North German Basin Margin: From Initial Triassic Salt Movement to Late Cretaceous-Cenozoic Remobilization, Tectonics, 39, e2019TC005927, https://doi.org/10.1029/2019TC005927, 2020.
Ahlrichs, N., Noack, V., Hübscher, C., Seidel, E., Warwel, A., and Kley, J.: Impact of Late Cretaceous inversion and Cenozoic extension on salt structure growth in the Baltic sector of the North German Basin, Basin Res., 34, 220–250, https://doi.org/10.1111/bre.12617, 2022.
Al Hseinat, M. and Hübscher, C.: Late Cretaceous to recent tectonic evolution of the North German Basin and the transition zone to the Baltic Shield/southwest Baltic Sea, Tectonophysics, 708, 28–55, https://doi.org/10.1016/j.tecto.2017.04.021, 2017.
Antonowicz, L., Iwanowska, E., and Rendak, A.: Tensional tectonics in the Pomeranian section of the TT Zone and the implications for hydrocarbon exploration, Geol. Q., 38, 289–306, 1994.
Babel Working Group: Deep Seismic Reflection/Refraction Interpretation of Crustal Structure along Babel Profiles A and B in the Southern Baltic Sea, Geophys. J. Int., 112, 325–343, https://doi.org/10.1111/j.1365-246X.1993.tb01173.x, 1993.
Bayer, U., Grad, M., Pharaoh, T. C., Thybo, H., Guterch, A., Banka, D., Lamarche, J., Lassen, A., Lewerenz, B., Scheck, M., and Marotta, A.-M.: The southern margin of the East European Craton: new results from seismic sounding and potential fields between the North Sea and Poland, Tectonophysics, 360, 301–314, https://doi.org/10.1016/S0040-1951(02)00359-1, 2002.
Beier, H. and Katzung, G.: Lithologie und Strukturgeologie des Altpaläozoikums in der Offshore-Bohrung G 14-1/86 (südliche Ostsee), Greifswalder Geowissenschaftliche Beiträge, 6, 327–345, 1999.
Berthelsen, A.: The Tornquist Zone northwest of the Carpathians: An intraplate pseudosuture, GFF, 120, 223–230, https://doi.org/10.1080/11035899801202223, 1998.
Botor, D., Mazur, S., Anczkiewicz, A. A., Dunkl, I., and Golonka, J.: Thermal history of the East European Platform margin in Poland based on apatite and zircon low-temperature thermochronology, Solid Earth, 12, 1899–1930, https://doi.org/10.5194/se-12-1899-2021, 2021.
Claerbout, J. F. and Doherty, S. M.: Downward continuation of moveout-corrected seismograms, Geophysics, 37, 741–768, https://doi.org/10.1190/1.1440298, 1972.
Dadlez, R., Narkiewicz, M., Stephenson, R. A., Visser, M. T. M., and van Wees, J.-D.: Tectonic evolution of the Mid-Polish Trough: modelling implications and significance for central European geology, Tectonophysics, 252, 179–195, https://doi.org/10.1016/0040-1951(95)00104-2, 1995.
Dallmeyer, R. D., Giese, U., Glasmacher, U., and Pickel, W.: First 40Ar/39Ar age constraints for the Caledonian evolution of the Trans–European Suture Zone in NE Germany, J. Geol. Soc., 156, 279–290, https://doi.org/10.1144/gsjgs.156.2.0279, 1999.
DEKORP-BASIN Research Group: Deep crustal structure of the Northeast German basin: New DEKORP-BASIN '96 deep-profiling results, Geology, 27, 55–58, https://doi.org/10.1130/0091-7613(1999)027<0055:DCSOTN>2.3.CO;2, 1999.
Erlström, M., Thomas, S. A., Deeks, N., and Sivhed, U.: Structure and tectonic evolution of the Tornquist Zone and adjacent sedimentary basins in Scania and the southern Baltic Sea area, Tectonophysics, 271, 191–215, https://doi.org/10.1016/S0040-1951(96)00247-8, 1997.
Franke, D., Gründel, J., Lindert, W., Meissner, B., Schulz, E., Zagora, I., and Zagora, K.: Die Ostseebohrung G 14–eine Profilübersicht, Z. Geol. Wissenschaft., 22, 235–240, 1994.
Gągała, Ł., Vergés, J., Saura, E., Malata, T., Ringenbach, J.-C., Werner, P., and Krzywiec, P.: Architecture and orogenic evolution of the northeastern Outer Carpathians from cross-section balancing and forward modelling, Tectonophysics, 532–535, 223–241, https://doi.org/10.1016/j.tecto.2012.02.014, 2012.
Graversen, O.: Upper Triassic–Lower Cretaceous seismic sequence stratigraphy and basin tectonics at Bornholm, Denmark, Tornquist Zone, NW Europe, Mar. Petrol. Geol., 21, 579–612, https://doi.org/10.1016/j.marpetgeo.2003.12.001, 2004.
Guterch, A., Grad, M., Janik, T., Materzok, R., Luosto, U., Yliniemi, J., Luck, E., Schulze, A., and Forste, K.: Crustal structure of the transition zone between Precambrian and Variscan Europe from new seismic data along LT-7 profile (NW Poland and eastern Germany), CR Acad. Sci. II A, 319, 1489–1496, 1994.
Hansen, M. and Poulsen, V.: Ekskursionsfører nr. 1, Geologi på Bornholm, VARV, København, 96 pp., ISBN 87-87624-05-2, 1977.
Harlan, W. S.: Regularization by model reparameterization, http://www.billharlan.com/papers/regularization.pdf (last access: 25 April 2024), 1995.
Hübscher, C.: Geophysical profiles during Maria S. Merian cruise MSM52, Inst. Für Geophys. Univ. Hamburg, https://doi.org/10.1594/PANGAEA.890870, 2018.
Hübscher, C., Ahlrichs, H., Allum, G., Behrens, T., Bülow, J., Krawczyk, C., Damm, V., Demir, Ü., Engels, M., Frahm, L., Grzyb, G., Hahn, B., Heyde, I., Juhlin, C., Knevels, K., Lange, G., Bruun Lydersen, I., Malinowski, M., Noack, V., Preine, J., Rampersad, K., Schnabel, M., Seidel, E., Sopher, D., Stakemann, Jo., and Stakemann, Ja.: BalTec – Cruise No. MSM52 – March 1 – March 28, 2016 – Rostock (Germany) – Kiel (Germany), Maria S. Merian-Berichte, MSM52, 46 pp., DFG Senatskommission für Ozeanographie, https://doi.org/10.2312/cr_msm52, 2017.
Janik, T., Wójcik, D., Ponikowska, M., Mazur, S., Skrzynik, T., Malinowski, M., and Hübscher, C.: Crustal structure across the Teisseyre-Tornquist Zone offshore Poland based on a new refraction/wide-angle reflection profile and potential field modelling, Tectonophysics, 828, 229271, https://doi.org/10.1016/j.tecto.2022.229271, 2022.
Jaworowski, K., Wagner, R., Modliski, Z., Pokorski, J., Sokołowski, J., and Sokołowski, A.: Marine ecogeology in semi-closed basin: case study on a threat of geogenic pollution of the southern Baltic Sea (Polish Exclusive Economic Zone), Geol. Q., 54, 267–288, 2010.
Karnkowski, P. H., Pikulski, L., and Wolnowski, T.: Petroleum geology of the Polish part of the Baltic region – an overview, Geol. Q., 54, 143–158, 2010.
Katzung, G.: The Caledonides at the southern margin of the East European Craton, Neues Jahrb. Geol. P.-A., 222, 3–53, https://doi.org/10.1127/njgpa/222/2001/3, 2001.
Katzung, G., Giese, U., Walter, R., and Winterfeld, C. V.: The Rügen Caledonides, northeast Germany, Geol. Mag., 130, 725–730, https://doi.org/10.1017/S0016756800021038, 1993.
Kramarska, R., Krzywiec, P., Dadlez, R., Jegliński, W., Papiernik, B., Przezdziecki, P., and Zientara, P.: Geological map of the Baltic Sea bottom without Quaternary deposits, Państwowy Instytut Geologiczny, Gdansk-Warszawa, 1999.
Krawczyk, C. M., Eilts, F., Lassen, A., and Thybo, H.: Seismic evidence of Caledonian deformed crust and uppermost mantle structures in the northern part of the Trans-European Suture Zone, SW Baltic Sea, Tectonophysics, 360, 215–244, https://doi.org/10.1016/S0040-1951(02)00355-4, 2002.
Krzywiec, P.: Contrasting tectonic and sedimentary history of the central and eastern parts of the Polish Carpathian foredeep basin – results of seismic data interpretation, Mar. Petrol. Geol., 18, 13–38, https://doi.org/10.1016/S0264-8172(00)00037-4, 2001.
Krzywiec, P.: Mid-Polish Trough inversion – seismic examples, main mechanisms, and its relationship to the Alpine-Carpathian collision, Stephan Mueller Spec. Publ. Ser., 1, 151–165, https://doi.org/10.5194/smsps-1-151-2002, 2002.
Krzywiec, P., Kramarska, R., and Zientara, P.: Strike-slip tectonics within the SW Baltic Sea and its relationship to the inversion of the Mid-Polish Trough – evidence from high-resolution seismic data, Tectonophysics, 373, 93–105, https://doi.org/10.1016/S0040-1951(03)00286-5, 2003.
Krzywiec, P., Kufrasa, M., Poprawa, P., Mazur, S., Koperska, M., and Ślemp, P.: Together but separate: decoupled Variscan (late Carboniferous) and Alpine (Late Cretaceous–Paleogene) inversion tectonics in NW Poland, Solid Earth, 13, 639–658, https://doi.org/10.5194/se-13-639-2022, 2022a.
Krzywiec, P., Stachowska, A., Grzybowski, Ł., Nguyen, Q., Słonka, Ł., Malinowski, M., Kramarska, R., Ahlrichs, N., and Huebscher, C.: The Late Cretaceous inversion of the Polish Basin and surrounding area – a current perspective based on seismic data, in: Cretaceous of Poland and of Adjacent Areas, 11th International Cretaceous Symposium, edited by: Jagt, J. W. M., Jagt-Yazykova, E., Walaszczyk, I., and Żylińska, A., Faculty of Geology, University of Warsaw, Warsaw, Poland, 9–23, 2022b.
Lassen, A., Thybo, H., and Berthelsen, A.: Reflection seismic evidence for Caledonian deformed sediments above Sveconorwegian basement in the southwestern Baltic Sea, Tectonics, 20, 268–276, https://doi.org/10.1029/2000TC900028, 2001.
Liboriussen, J., Ashton, P., and Tygesen, T.: The tectonic evolution of the Fennoscandian Border Zone in Denmark, Tectonophysics, 137, 21–29, https://doi.org/10.1016/0040-1951(87)90310-6, 1987.
Mazur, S., Scheck-Wenderoth, M., and Krzywiec, P.: Different modes of the Late Cretaceous–Early Tertiary inversion in the North German and Polish basins, Int. J. Earth Sci., 94, 782–798, https://doi.org/10.1007/s00531-005-0016-z, 2005.
Mazur, S., Mikolajczak, M., Krzywiec, P., Malinowski, M., Buffenmyer, V., and Lewandowski, M.: Is the Teisseyre-Tornquist Zone an ancient plate boundary of Baltica?, Tectonics, 34, 2465–2477, https://doi.org/10.1002/2015TC003934, 2015.
Mazur, S., Mikolajczak, M., Krzywiec, P., Malinowski, M., Lewandowski, M., and Buffenmyer, V.: Pomeranian Caledonides, NW Poland – A collisional suture or thin-skinned fold-and-thrust belt?, Tectonophysics, 692, 29–43, https://doi.org/10.1016/j.tecto.2016.06.017, 2016.
Nguyen, Q., Malinowski, M., Kramarska, R., Kaulbarsz, D., Mil, L., and Hübscher, C.: Gas-Escape features along the Trzebiatów fault offshore Poland: Evidence for a leaking petroleum system, Mar. Petrol. Geol., 156, 106431, https://doi.org/10.1016/j.marpetgeo.2023.106431, 2023.
Pan, Y., Seidel, E., Juhlin, C., Hübscher, C., and Sopher, D.: Inversion tectonics in the Sorgenfrei–Tornquist Zone: insight from new marine seismic data at the Bornholm Gat, SW Baltic Sea, GFF, 144, 71–88, https://doi.org/10.1080/11035897.2022.2071335, 2022.
Pharaoh, T. C.: Palaeozoic terranes and their lithospheric boundaries within the Trans-European Suture Zone (TESZ): a review, Tectonophysics, 314, 17–41, https://doi.org/10.1016/S0040-1951(99)00235-8, 1999.
Pietsch, K. and Krzywiec, P.: Application of seismic methods for hydrocarbon exploration within the Devonian and Carboniferous series of the Western Pomerania (Białogard-Jamno area), Oil and Gas News from Poland, 6, 175–186, 1996.
Piske, J., Rasch, H. J., Neumann, E., and Zagora, K.: Geologischer Bau und Entwicklung des Präperms der Insel Rügen und des angrenzenden Seegebietes, Z. Geol. Wissenschaft., 22, 211–226, 1994.
Podhalańska, T. and Modliński, Z.: Outline of the lithology and depositional features of the lower Paleozoic strata in the Polish part of the Baltic region, Geol. Q., 54, 109–121, 2010.
Pokorski, J.: Geological section through the lower Paleozoic strata of the Polish part of the Baltic region, Geol. Q., 54, 123–130, 2010.
Ponikowska, M., Stovba, S. M., Mazur, S., Malinowski, M., Krzywiec, P., Nguyen, Q., and Hübscher, C.: Crustal-scale pop-up structure at the junction of two continental-scale deformation zones in the southern Baltic Sea, Tectonics, 43, e2023TC008066, https://doi.org/10.1029/2023TC008066, 2024.
Rempel, H.: Erdölgeologische Bewertung der Arbeiten der Gemeinsamen Organisation Petrobaltic im deutschen Schelfbereich, Geologisches Jahrbuch. Reihe D. Mineralogie, Petrographie, Geochemie, Lagerstättenkunde, 99, 3–32, 1992.
Schlüter, H., Best, G., Jürgens, U., and Binot, F.: Interpretation reflexionsseismischer Profile zwischen baltischer Kontinentalplatte und kaledonischem Becken in der südlichen Ostsee – erste Ergebnisse, Zeitschrift der Deutschen Geologischen Gesellschaft, 148, 1–32, 1997.
Schlüter, H. U., Jürgens, U., Binot, F., and Best, G.: The importance of geological structures as natural sources of potentially hazardous substances in the southern part of the Baltic Sea, Z. Angew. Geol., 44, 26–32, 1998.
Seidel, E., Meschede, M., and Obst, K.: The Wiek Fault System east of Rügen Island: origin, tectonic phases and its relationship to the Trans-European Suture Zone, Geol. Soc. Lond. Spec. Publ., 469, 59–82, https://doi.org/10.1144/SP469.10, 2018.
Smit, J., van Wees, J.-D., and Cloetingh, S.: Early Carboniferous extension in East Avalonia: 350 My record of lithospheric memory, Mar. Petrol. Geol., 92, 1010–1027, https://doi.org/10.1016/j.marpetgeo.2018.01.004, 2018.
Sopher, D., Erlström, M., Bell, N., and Juhlin, C.: The structure and stratigraphy of the sedimentary succession in the Swedish sector of the Baltic Basin: New insights from vintage 2D marine seismic data, Tectonophysics, 676, 90–111, https://doi.org/10.1016/j.tecto.2016.03.012, 2016.
Thomas, S. A., Sivhed, U., Erlström, M., and Seifert, M.: Seismostratigraphy and structural framework of the SW Baltic Sea, Terra Nova, 5, 364–374, https://doi.org/10.1111/j.1365-3121.1993.tb00270.x, 1993.
Thybo, H.: Crustal structure and tectonic evolution of the Tornquist Fan region as revealed by geophysical methods, B. Geol. Soc. Denmark, 46, 145–160, https://doi.org/10.37570/bgsd-1999-46-12, 1999.
Thybo, H.: Crustal structure along the EGT profile across the Tornquist Fan interpreted from seismic, gravity and magnetic data, Tectonophysics, 334, 155–190, https://doi.org/10.1016/S0040-1951(01)00055-5, 2001.
Torsvik, T. H. and Rehnström, E. F.: The Tornquist Sea and Baltica–Avalonia docking, Tectonophysics, 362, 67–82, https://doi.org/10.1016/S0040-1951(02)00631-5, 2003.
van Wees, J.-D., Stephenson, R. A., Ziegler, P. A., Bayer, U., McCann, T., Dadlez, R., Gaupp, R., Narkiewicz, M., Bitzer, F., and Scheck, M.: On the origin of the Southern Permian Basin, Central Europe, Mar. Petrol. Geol., 17, 43–59, https://doi.org/10.1016/S0264-8172(99)00052-5, 2000.
Vejbaek, O. V., Stouge, S., and Damtoft Poulsen, K.: Palaeozoic tectonic and sedimentary evolution and hydrocarbon prospectivity in the Bornholm area, https://www.osti.gov/etdeweb/biblio/49079 (last access: 25 April 2024), 1994.
Verschuur, D. J.: Seismic Multiple Removal Techniques: Past, present and future (EET 1), Earthdoc, https://doi.org/10.3997/9789073834965, 2013.