the Creative Commons Attribution 4.0 License.
the Creative Commons Attribution 4.0 License.
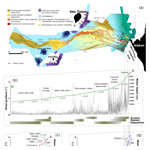
The Ogooue Fan (offshore Gabon): a modern example of deep-sea fan on a complex slope profile
Salomé Mignard
Thierry Mulder
Philippe Martinez
Thierry Garlan
The effects of changes in slope gradient on deposition processes and architecture have been investigated in different deep-sea systems both in modern and ancient environments. However, the impact of subtle gradient changes (< 0.3∘) on sedimentary processes along deep-sea fans still needs to be clarified. The Ogooue Fan, located in the northeastern part of the Gulf of Guinea, extends over more than 550 km westwards of the Gabonese shelf and passes through the Cameroon volcanic line. Here, we present the first study of acoustic data (multibeam echosounder and 3.5 kHz, very high-resolution seismic data) and piston cores covering the deep-sea part of this West African system. This study documents the architecture and sedimentary facies distribution along the fan. Detailed mapping of near-seafloor seismic-reflection data reveals the influence of subtle slope gradient changes (< 0.2∘) along the fan morphology. The overall system corresponds to a well-developed deep-sea fan, fed by the Ogooue River sedimentary load, with tributary canyons, distributary channel–levee complexes and lobe elements. However, variations in the slope gradient due to inherited salt-related structures and the presence of several seamounts, including volcanic islands, result in a topographically complex slope profile including several ramps and steps. In particular, turbidity currents derived from the Gabonese shelf deposit cross several interconnected intra-slope basins located on the low gradient segments of the margin (< 0.3∘). On a higher gradient segment of the slope (0.6∘), a large mid-system valley developed connecting an intermediate sedimentary basin to the more distal lobe area. Distribution and thickness of turbidite sands is highly variable along the system. However, turbidite sands are preferentially deposited on the floor of the channel and the most proximal depositional areas. Core description indicates that the upper parts of the turbidity flows, mainly composed of fine-grained sediments, are found in the most distal depocenters.
- Article
(11215 KB) - Full-text XML
- BibTeX
- EndNote
Deep-sea fans are depositional sinks that host stratigraphic archives of Earth history and environmental changes (Clift and Gaedicke, 2002; Fildani and Normark, 2004; Covault et al., 2010, 2011) and are also important reservoirs of natural resources (Pettingill and Weimer, 2002). Therefore, considerable attention has been given to the problems of predicting architectures and patterns of sedimentary facies distribution in submarine fans. Early models concerning the morphologies of these systems described submarine fans as cone-like depositional areas across unconfined basin floors of low relief and with a gentle slope gradient (Shepard and Emery, 1941; Shepard, 1951; Dill et al., 1954; Menard, 1955; Heezen et al., 1959). However, studies of outcrops (Kane et al., 2010) and modern seabed datasets (Stevenson et al., 2013; Kneller, 1995) showed that topographic complexity across the receiving basin can strongly influence the organization of architectural elements of submarines fans (Normark et al., 1983; Piper and Normark, 2009). A wide range of geometries and architectural features due to topographic obstacles has been described in the literature. Among these features are ponded and intra-slope mini basins due to three-dimensional confinement (Prather, 2003; Prather et al., 2017; Sylvester et al., 2015) or tortuous corridors created by topographic barriers (Smith, 2004; Hay, 2012). Spatial changes in slope gradients are also important as they cause gravity flows to accelerate or decelerate along the slope (Normark and Piper, 1991; Mulder and Alexander, 2001) allowing the construction of several connected depocenters and sediment bypass areas (Smith, 2004; Deptuck et al., 2012; Hay, 2012). These stepped slopes have been described along modern systems such as the Niger Delta (Jobe et al., 2017), the Gulf of Mexico (Prather et al., 1998, 2017) or offshore Angola (Hay, 2012), but also in ancient systems such as the Annot Sandstone Formation (Amy et al., 2007; Salles et al., 2014), the Karoo Basin (Spychala et al., 2015; Brooks et al., 2018) or the Lower Congo Basin (Ferry et al., 2005).
On stepped slopes where structural deformation is very slow, sediment erosion and deposition are the dominant processes that control the short-term evolution of slope. In these systems, the slope gradient variations play a key role and studies have shown that subtle gradient changes (< 0.3∘) can have an important impact on flow velocity and consequently deep-sea fan organization (e.g., Kneller, 1995; Kane et al., 2010; Stevenson et al., 2013). Even though some of these systems have already been described, the impact of subtle changes in slope gradient on deep-sea fan organization still needs to be better understood in order to extend our knowledge on terrestrial sediments routing and on the potential for reservoir deposits in stepped slope settings.
The modern Ogooue Fan provides a new large-scale example of the influence of gradient changes on deep-sea sediment routing. This system, which results from the sediment discharge of the Ogooue River, is the third largest system of the Gulf of Guinea after the Congo and the Niger fans (Séranne and Anka, 2005). However, in contrast to these two systems that have been the focus of many studies (Droz et al., 1996, 2003; Babonneau et al., 2002; Deptuck et al., 2003, 2007), the Quaternary sediments of the Gabon passive margin have not been studied, especially in its deepest parts (Bourgoin et al., 1963; Giresse, 1969; Giresse and Odin, 1973). The regional survey of the area by the SHOM (Service Hydrographique et Océanographique de la Marine) in 2005 and 2010, during the OpticCongo and MOCOSED cruises, provided the first extensive dataset of the Ogooue deep-sea fan, from the continental shelf to the abyssal plain.
The objective of this paper is to document the overall fan morphology and to link its evolution with the local changes in slope gradients or topographic obstacles present in the depositional area. This study contributes to the understanding of the impact of subtle slope gradient changes on a whole deep-water system. This study can be used to develop predictive models of sedimentary facies distribution for systems located on a stepped slope with low gradient changes (< 0.5∘) and to better constrain sand deposits.
The continental margin of the Gulf of Guinea formed during the rifting that occurred within Gondwana in Neocomian to lower Aptian times. Syn-rift deposits are buried by mid–Late Cretaceous transgressive sedimentary rocks consisting initially of evaporites, which have created salt-related deformations of the margin sediments, followed by platform carbonates (Cameron and White, 1999; Mougamba, 1999; Wonham et al., 2000; Séranne and Anka, 2005). Since the Late Cretaceous, the West African margin has recorded clastic sedimentation fed by the denudation of the African continent (Séranne and Anka, 2005). Different periods of major uplift and canyon incisions occurred from Eocene to lower Miocene times (Rasmussen, 1996; Wonham et al., 2000; Séranne and Anka, 2005). The sediment depocenters were located basinward of the main rivers, such as the Niger, Congo, Ogooue or Orange River forming vast and thick deep-sea fans (Mougamba, 1999; Séranne and Anka, 2005; Anka et al., 2009).
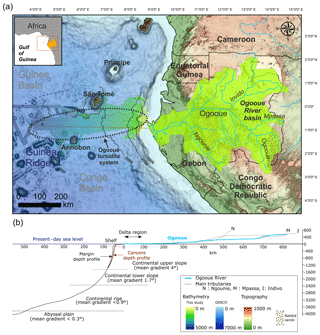
Figure 1(a) The Ogooue sedimentary system from source (river and drainage basin) to sink (Quaternary turbidite fan). (b) Channel depth profile of the Ogooue River (blue) and its main tributaries (grey) and mean depth profile along the Gabonese margin.
The Ogooue Fan is located in the northeastern part of the Gulf of Guinea on the Gabonese continental slope. The fan developed on the Guinea Ridge, which separates the two deep Congo and Guinea basins. This region is notably characterized by the presence of several volcanic islands belonging to the Cameroon volcanic line (CVL) associated with rocky seamounts (Fig. 1a). Geophysical studies of the volcanic line suggest that the volcanic alignment is related to a deep-mantle hot line (Déruelle et al., 2007). All the volcanoes of the CVL have been active for at least 65 Myr (Lee et al., 1994; Déruelle et al., 2007). Ar/Ar dates performed on São Tomé and Annobón volcanic rocks proved the activity of theses volcanic island over much of the Pleistocene (Lee et al., 1994; Barfod and Fitton, 2014). The MOCOSED 2010 cruise revealed that numerous mud volcanoes were associated with the toe of the slopes of the volcanic islands (Garlan et al., 2010). They form small topographic highs on the seafloor (< 20 m high and 100 m in diameter) and show active gas venting (Garlan et al., 2010).
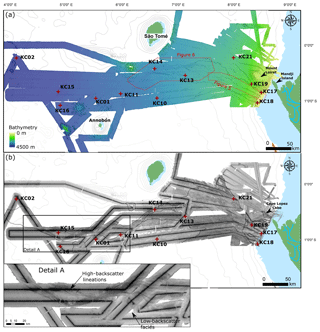
Figure 2(a) Detailed bathymetric map of the Ogooue Fan, based on the multibeam echosounder data of the Optic Congo2005 and MOCOSED2010 surveys. (b) Acoustic imagery of the Ogooue Fan (high backscatter: dark tones; low backscatter: light tones). Detail A: close-up of the deepest part of the Ogooue Fan. Red crosses: location of the studied cores.
The Quaternary Ogooue Fan extends westwards over 550 km through the CVL. Overall, the modern slope profile is concave upward, similar to other passive margins, e.g., eastern Canada margin and north Brazilian margin (Covault et al., 2012). The mean slope gradient shallows from 7∘ on the very upper slope to < 0.3∘ in the abyssal plain (Fig. 1b). The Gabonese continental shelf, which is relatively narrow, can be divided into two subparts: the south Gabon margin presenting a SE–NW orientation and the north Gabon margin presenting a SW–NE orientation. The southern part of the margin is characterized by the presence of numerous parallel straight gullies oriented perpendicular to the slope (Séranne and Nzé Abeigne, 1999; Lonergan et al., 2013). On the north Gabon margin, the area located between 1∘00 S and Mandji Island is incised by several canyons that belong to the modern Ogooue Fan (Fig. 2a). North of Mandji Island, the seafloor reveals numerous isolated pockmarks as well as sinuous trains of pockmarks. These features are interpreted as the results of fluid migration from shallow buried channels (Gay et al., 2003; Pilcher and Argent, 2007).
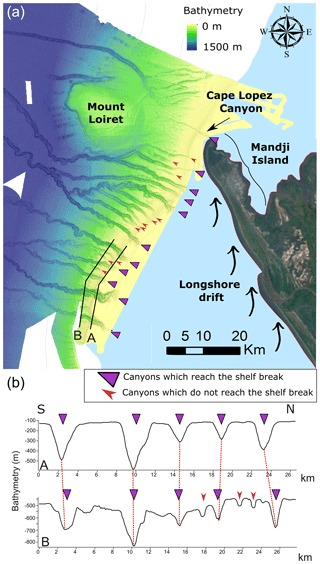
Figure 3(a) Close-up view of the Gabon shelf and canyons ramp. Bathymetry is from the Optic Congo2005 and MOCOSED2010 surveys; satellite view is from Google Earth. (b) Two bathymetric profiles across the canyons showing the two types of canyons which are present along the Gabonese slope.
The Ogooue Fan is supplied by the sedimentary load of the Ogooue River, which is third largest African freshwater source in the Atlantic Ocean (Mahé et al., 1990). Despite the relatively small size of the Ogooue River basin (215 000 km2), the river mean annual discharge reaches 4700 m3 s−1 due to the wet equatorial climate (Lerique et al., 1983; Mahé et al., 1990). The Ogooue River flows on a low slope gradient in a drainage basin covered essentially with thick lateritic soils that developed over the Congo craton and Proterozoic formations related to Precambrian orogenic belts (Séranne et al., 2008). The estuary area includes several lakes which trap coarse sediments (Fig. 1b) (Lerique et al., 1983) and contribute to the dominant muddy composition of the particle load of the Ogooue River that is estimated between 1 and 10 MT (megaton) yr−1. (Syvitski et al., 2005). The limited portion of sand particles in the river originates mainly from the erosion of the poorly lithified Batéké Sands located on a 550–750 m high perched plateau that forms the easternmost boundary of the Ogooue watershed (Séranne et al., 2008) (Fig. 1a). On the shelf, recent fluviatile deposits consist of fine-grained sediments deposited at the mouth of the Ogooue River (Giresse and Odin, 1973). The wave conditions on the Gabonese coast are characterized by a predominant direction from south to southwest. Reflection of these southwesterly swells causes coastal sediments to be transported northward (Biscara et al., 2013). Sedimentary transport linked to longshore drift ranges between 300 000 and 400 000 m3 yr−1 (Bourgoin et al., 1963) and is responsible for the formation of Mandji Island, a sandy spit 50 km long located at the northern end of the Ogooue Delta (Fig. 3). Except for the Cape Lopez Canyon, located just west of Mandji Island with the canyon head in only 5 m water depth (Biscara et al., 2013), the Ogooue Fan is disconnected from the Ogooue Delta during the present-day high sea level (Fig. 3).
The bathymetry and acoustic imagery of the studied area result from the multibeam echosounder (Seabat 7150) surveys conducted onboard the R/V Pourquoi Pas? and Beautemps-Beaupré during the MOCOSED 2010 and OpticCongo 2005 cruises (Mouscardes, 2005; Guillou, 2010) (Fig. 2). The multibeam backscatter data (Fig. 2b) have been used to characterize the distribution of sedimentary facies along the margin. Changes in the backscatter values correspond to variations in the nature, the texture and the state of sediments and/or the seafloor morphology (Unterseh, 1999; Hanquiez et al., 2007). On the multibeam echosounder images, lighter areas indicate low acoustic backscatter and darker areas indicate high backscatter. Five main backscatter types are identified on the basis of backscatter values and homogeneity (Fig. 4). Facies A is a homogenous low-backscatter facies, Facies B is a low-backscatter heterogeneous facies and Facies C is a medium-backscatter facies characterized by the presence of numerous higher-backscatter patches. Facies D and E are high- and very high-backscatter facies, respectively. High-backscatter lineations are present within Facies D.
A total of 4500 km of 3.5 kHz seismic lines were collected in the area of the Ogooue Fan during the MOCOSED 2010 cruise and 470 km during the Optic Congo 2005 cruise (iXblue ECHOES 3500 T7). These data were used to analyze the near-surface deposits. The dataset covers the shelf edge, the slope and the abyssal plain. In this study, the 3.5 kHz echofacies have been classified according to Damuth's methodology (Damuth, 1975, 1980a; Damuth and Hayes, 1977) based on acoustic penetration and continuity of bottom and sub-bottom reflection horizons, micro-topography of the seafloor, and the presence of internal structures.
The 12 Küllenberg cores presented here were collected during the cruise MOCOSED 2010. Five of these cores have already been presented in Mignard et al. (2017) (Table 1). Visual descriptions of the cores discriminated between the dominant grain size (clay, silty clay, silt and fine sand) and vertical successions of sedimentary facies. Thin slabs were collected for each split core section and X-ray radiographed using a SCOPIX digital X-ray imaging system (Migeon et al., 1998). Subsamples were regularly taken in order to measure carbonate content using a gasometric calcimeter and grain size using a Malvern Mastersizer S.
4.1 Sedimentary facies
The classification into five sedimentary facies used here is based on photography and X-ray imagery, grain size analyses, and CaCO3 content (Fig. 5, Table 2). Interpretation of these facies is based on the comparison with previous sedimentary facies classifications such as Stow and Piper (1984), Pickering et al. (1986), and Normark and Damuth (1997).
4.2 Fan morphology
Analysis of the seafloor data (bathymetry and acoustic imagery) reveals the different domains of the Ogooue sedimentary system and the different architectural features of the Ogooue Fan (Fig. 6).
The Gabon continental shelf is relatively narrow, decreasing in width from 60 to 5 km toward Mandji Island (Fig. 3). The slope is characterized by two main topographic features: (1) Mount Loiret, a guyot located just west of Mandji Island, which forms a bathymetric obstacle on the upper slope, and (2) a ramp of several tributary canyons located south of Mount Loiret (Fig. 3). This ramp is composed of several wide and deep canyons (several hundreds of meters deep and 2–3 km wide near the canyon head), with a “V-shaped” morphology and the heads of which reach the shelf break. Several thinner and shallower incisions are located between these deep canyons. They are less than 100 m deep and 1 km wide, and their heads are located between 200 and 400 m water depth (Fig. 3). The continental shelf and the slope present low-backscatter values except for the canyons, which correspond to very high backscatter values (Fig. 4).
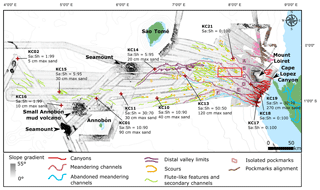
Figure 6Interpreted gradient-shaded map of the Ogooue Fan showing the main features of the fan. A, B, C, D, E and F are the six main channels discussed in the text. The sand ∕ shale ratio of the cores are shown (Sa:Sh) as well as the maximum sand-bed thickness in each core (max sand). A close-up view of the red rectangle is presented in Fig. 8.
The transition between the continental slope and the continental rise, between 1200 and 1500 m water depth, is marked by a decrease in the slope gradient from a mean value of 2.3 to 0.9∘. At this water depth, several canyons merge to form five sinuous channels (B to F in Fig. 6). These channels appear with a higher backscatter values than the surrounding seafloor (Fig. 4). These sinuous subparallel channel–levee complexes extend down to 2200 m water depth with a general course oriented toward the northwest (Figs. 6 and 7). At 2200 m water depth, the southernmost channel (channel F in Fig. 6) changes its path toward the southwest.
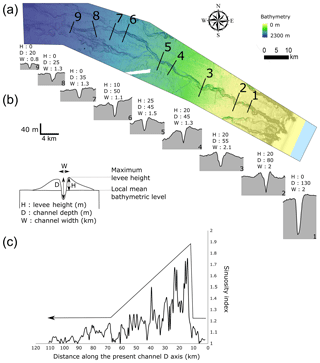
Figure 7(a) Detailed bathymetric map of channel D (location in Fig. 2), (b) serial bathymetric profiles showing the evolution of the channel–levees along the slope and (c) sinuosity down the channel D measured along 2 km channel segments.
The sinuosity of these channels decreases westward. Channel D sinuosity has been calculated over 2 km long segments (Fig. 7c). It is less than 1.1 along the first 13 km corresponding to the canyon part. From 13 to 40 km the mean sinuosity is 1.4 and then decreases to less than 1.2 between 40 and 90 km from the head. Finally, the most distal part of the channel, from 90 km from the head, is very straight with a sinuosity index lower than 1.1 (Fig. 7c).
Downslope, in the central part of the system, the seafloor located between 2200 and 2500 m water depth presents numerous erosional features including scours, lineations and smaller, subsidiary channels, corresponding to channels with no headward connection with an obvious feeder system according to Masson et al. (1995) (Fig. 8). These erosional features appear on a very gentle slope area (0.3∘) characterized by a heterogeneous medium-backscatter facies (Fig. 4). At 2500 m water depth, just south of the São Tomé Island, the head of a large, 100 km long, mid-system valley appears (Fig. 9). This valley can be subdivided into two parts of approximately equal length with two different orientations. The upper part of the valley is oriented E–W, whereas the lower part is oriented NE–SW. This directional change is due to the presence of a rocky seamount located north of the valley and which deflects its course. The upper part of the valley is up to 15 km wide with numerous erosional scars and terraces on its flanks. The valley bottom is characterized by very high backscatter values and small internal erosion channels. Downstream, the valley becomes narrower with a “U” shape (Fig. 9, profile 5). Its flanks appear regular, with no scar of down-flank mass deposits. The depth of the valley decreases from 60 m in its central part to only 10 m near its mouth. The area located south of the mid-system valley is characterized by a heterogeneous low-backscatter facies. Some erosional features and subsidiary channels are present but scarce.
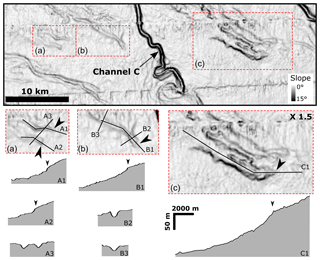
Figure 8Close-up view of the gradient-shaded map showing erosional lineations (a and b) and amalgamated scours (c) in the central part of the system (location in Fig. 6).
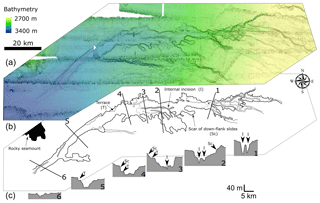
Figure 9(a) Detailed bathymetric map of the mid-system valley of the Ogooue Fan between 2700 and 3400 m water depth. (b) Interpretation of the main morphological features of the valley. (c) Six transverse profiles of the mid-system valley extracted from the bathymetry data (Sc: scar of down-flank slides; I: internal incision; T: terrace).
West of the mid-system valley outlet, the seafloor is very flat and shows only subtle morphological variations except for local seamounts. A few channel-like, narrow elongated depressions (maximum 10 m deep) presenting high backscatter values can be identified. These lineations are restricted to a long tongue of high backscatter at the mouth of the valley (Fig. 2b, Detail A). This tongue is globally oriented E–W at the exit of the mid-system valley and then deflects toward the NW at 3700 m water depth, following the steepest slope.
North of Mount Loiret, the upper slope presents a lower slope gradient compared to the south part and is characterized by the presence of numerous linear pockmark trains on the upper part and pockmarks fields on the lower part. This whole area has a very low and homogenous reflectivity. Traces of active sedimentation on this part of the margin are only visible in association with the Cape Lopez Canyon (Fig. 3). Cape Lopez Canyon terminates at 650 m water depth at an abrupt decrease in slope gradient (from more than 1.7 to 0.6∘) caused by the presence of Mount Loiret (Fig. 10). This canyon is associated with a small intra-slope lobe located just northeast of Mount Loiret and referred as the Cape Lopez lobe (Fig. 10) (Biscara et al., 2011). This northern system continues basinward with channel A, the head of which is located in the vicinity of the Cape Lopez lobe. At 2200 m water depth, channel A ends and its mouth is associated on the backscatter map with a fan-shaped area of very high reflectivity, which is associated with some subsidiary channels and erosional marks (Fig. 4).
4.3 Echofacies classification and distribution
The main echofacies have been distinguished from each other on the profiles based on amplitude, frequency and geometry of the reflections (Fig. 11). They have been grouped into five main classes: (I) bedded, (II) bedded-rough, (III) rough, (IV) transparent and (V) hyperbolic. Most transitions between echofacies are gradual.
The echofacies of the edge of the Gabonese shelf consists of transparent echofacies IV (Fig. 11). North of Mount Loiret, the continental slope presents bedded echofacies I. At 1500 m, which corresponds to an increase in the slope gradient, echofacies transforms into echofacies I'. South of Mount Loiret, echofacies II and II' dominate on the continental slope.
The echomapping of the continental rise reveals the presence of different facies. The central part, just upstream of the mid-system valley, is characterized by rough echofacies III. Some large channels are marked by hyperbolic facies. South of the mid-system valley, facies II dominates. Echofacies IV is present in two main areas on the continental rise where they form two lobe-shaped zones: one on the northern part, following the limits of the high-reflectivity area located at the mouth of channel A; the second in the southern part of the system in association with channel F.
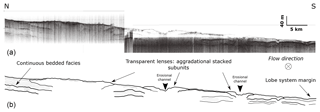
Figure 12(a) Transverse 3.5 kHz very high-resolution seismic line and (b) line drawing in the upper distal lobe area; see Fig. 11 for location of the line.
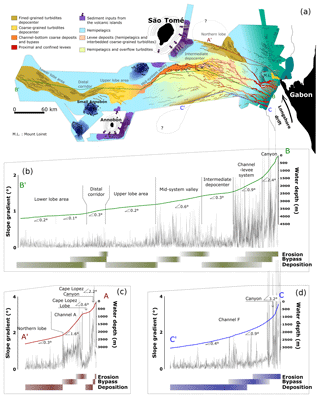
Figure 13(a) Synthetic map showing the architecture and the recent sedimentary processes of the Ogooue Fan determined by imagery and echofacies mapping. (b, c, d) Longitudinal profiles from the bathymetric data along the central, northern and southern part of the Ogooue Fan and slope gradient (in degree, measured every 100 m). The differences in slope gradient along the transects are associated with the main sedimentary processes encountered along the slope.
In the abyssal plain, the area of the elongated tongue noticeable on the backscatter data presents different echofacies. Based on the 3.5 kHz profiles, it can be subdivided into two main domains. The upstream part, at the outlet of the mid-system valley, is characterized by multiple aggradational stacked transparent subunits from 10 to 30 m thick, which are visible on the seismic lines (Fig. 12). The downstream part is characterized by echofacies (II) associated with hyperbolic echofacies (V).
At the edge of this tongue, high-penetration bedded facies (I) is dominant. Facies V' forms some patches on the seafloor and corresponds to seafloor mounts.
Facies V and IV are also present and form lenses around the island of São Tomé and Annobón.
Based on previous studies and core samples, we speculate on the following links between echofacies, type of sediments and associated depositional processes.
-
Bedded facies (I, I') are commonly associated with alternating sandy and silty beds (Damuth, 1975, 1980a; Pratson and Laine, 1989; Pratson and Coakley, 1996; Loncke et al., 2009) or with hemipelagic sedimentation when associated with very low reflectivity. This is confirmed by facies description of cores KC16 and KC02 (Gaullier and Bellaiche, 1998).
-
Rough and bedded-rough facies (II, II', III), as described in Loncke et al. (2009), are attributed to coarse-grained turbidite (Damuth, 1975; Damuth and Hayes, 1977). Damuth and Hayes (1977) have shown that a quantitative relationship exists between the relative abundance of coarse sediment in the upper few meters of the seafloor and the roughness of the echo-types. Rough echofacies characterized areas that contain the highest concentrations of coarse grains, like lobe areas, whereas bedded-rough facies contain little coarse sediments. Core KC10 and KC15, collected in an area of facies II, indicates the alternation of clayey and sandy layers but with a predominance of fine-grained sediments (Fig. 5).
-
Transparent facies (IV) commonly corresponds to structureless deposits due to mass-flow processes such as debris flows (Embley, 1976; Jacobi, 1976; Damuth, 1980a, b, 1994), but it can also characterize basinal fine-grained turbidites (Cita et al., 1984; Tripsanas et al., 2002). In this study transparent facies is also associated with fine-grained, structureless, terrigenous sedimentation of the shelf (Core KC18).
-
Hyperbolic facies (V, V') is linked to the degree of roughness of the seafloor topography. Large, irregular hyperbolae (V') are generally associated with abrupt topographies such as seamounts or canyons and deep channels. Small regular hyperbolae (V) are commonly associated with deposits generated by debris flow (Damuth, 1980a, b, 1994).
5.1 Sedimentary processes along the fan
The Ogooue Fan could be classified as a delta-fed passive margin deep-sea submarine fan according to Reading and Richards (1994). However, analysis of subsurface data (bathymetry, acoustic imagery and 3.5 kHz echocharacters) reveals a great variability of sediment processes in the different domains of the margin, controlled by variations in slope gradient and the presence of seamounts (Fig. 13a).
5.1.1 Upslope area and canyons system
Cores collected in the upslope area (KC18 and KC17) show mostly hemipelagic sediments with a very low carbonate content. This reflects significant detrital flux associated with proximity to the Ogooue platform and the influence of the Ogooue river plume. Erosional processes are also active on the upper part of the slope as indicated by the presence of numerous tributary canyons (Fig. 3). Based on the comparison of the canyon depths, widths and head positions, we observe the existence of two types of canyons as described in Jobe et al. (2011) along the Equatorial Guinea margin. The canyons which present a deep (> hundreds of meters deep) V shape and which indent the shelf edge are type I canyons (sensu Jobe et al., 2011), whereas the shallower canyons (< 100 m deep) with a U shape and which do not indent the shelf are type II canyons (sensu Jobe et al., 2011). The difference between these two types of canyons indicates different initiation and depositional processes. Type I canyons are commonly associated with high sediment supply and the canyons' initiation and morphology are controlled by frequent sand-rich erosive turbidity currents (Field and Gardner, 1990; Pratson et al., 1994; Pratson and Coakley, 1996; Weaver et al., 2000; Bertoni and Cartwright, 2005; Jobe et al., 2011). Core KC19 collected down of a type I canyon shows two several-meters thick sandy successions corresponding to top-cut-out Bouma sequences (Ta) interbedded with the upper slope hemipelagites. These sandy turbidites, which are the thickest sand beds recorded in all the cores (Fig. 6), indicate the occurrence of high-density turbidity currents flowing down this canyon. In contrast, type II canyons are found in areas of low sediment supply. Their initiation is attributed to retrogressive sediment failures and subsequent headward erosion (Shepard, 1981; Twichell and Roberts, 1982; Stanley and Moore, 1983). The evolution of these canyons is controlled by fine-grained sedimentation: hemipelagic deposition and dilute turbidity currents that can be carried over the shelf into the canyon heads. These sedimentary processes do not cause significant erosion in the canyons (Thornton, 1984).
North of Mount Loiret, the fine-grained sedimentation has completely infilled several type II canyons. The fluid migration from the previously deposited coarse-grained sediments inside the paleo-canyons has created sinuous trains of pockmarks. These pockmarks have been previously described in Pilcher and Argent (2007). Variations in the localization of coarse-grained sediment supplies play a key role on the development of the two types of canyons. Along the central Gabonese shelf, the very recent development of Mandji Island 3000 years BP (Giresse and Odin, 1973; Lebigre, 1983) concentrated most of the coarse sediments near Cape Lopez and favored the construction of the presently active Cape Lopez type I canyon (Biscara et al., 2013).
5.1.2 Channel system
The transition from deep canyons to sinuous channels with levees is related to a decrease in slope gradient from the continental slope (> 2∘) to the continental rise (< 1∘) that slows turbidity currents and reduces their erosional power. The external levees of the four central channels (B, C, D and E in Fig. 2) show high reflectivity compared to the surrounding seafloor, which indicates a different sedimentological nature. This suggests that deposition occurs on the low-development external levees (25 m maximum levee height for channel D; Fig. 7) due to turbidity current overspills. External levee deposits have been sampled by core KC13, which shows numerous turbidites made up of centimeter-thick, normally graded, parallel or ripple cross-laminated of silt and fine sands (Fig. 5). In their axial part, these channels are mainly erosive (Normark et al., 1993) as indicated by their deep incision in the seafloor: on average 70 m deep for channel D and 90 m deep for channel A (Figs. 7 and 10) below the associated levees, when present. This feature is similar to the modern Congo Channel (Babonneau et al., 2002) and is opposed to the morphology of aggrading channels (such as the Amazon Channel) where the thalweg is perched above the base of the levee system (Damuth, 1995). This entrenched morphology prevents extensive overflow of turbidity currents and is the probable cause of low development of external levees and limits channel avulsion. It has been proposed for the Congo Channel that the entrenched morphology of the channel confines the flow and maintains a high velocity. The high velocity of the flow enables the sediments to be transported to very distant areas (Babonneau et al., 2002).
Several studies have documented that sinuosity of submarine channels increases with time (Peakall et al., 2000; Babonneau et al., 2002; Deptuck et al., 2003, 2007; Kolla, 2007). The sinuous upper parts of the channels (1.3 < sinuosity < 1.75 for channel D; Fig. 7c) have consequently undergone a long history whereas the distal straighter parts of the channels are at a more immature stage. Moreover, the height of the external levees and the depth of the channels both decrease in the lower parts of the channel system (Fig. 7). These morphological changes are due to a slope gradient decrease (< 0.5∘ from transect 6 along channel D; Fig. 7) that progressively slows down the flow velocity and reduces the erosional power of the turbidity current. Simultaneously, the deposition of fine particles by spilling of the upper part of the flow on the external levees leads to a progressive decrease in the fine-grained fraction transported by the channelized flows (Normark et al., 1993; Peakall et al., 2000).
At 2200 m water depth, the appearance of numerous erosional features such as isolated and amalgamated spoon–shaped scours (Fig. 8 C1), erosional lineations and subsidiary channels with limited surface expression (10–2 m deep, Fig. 8 B2, B3) are characteristic of the channel lobe transition zone (Fig. 8) (Kenyon et al., 1995; Wynn et al., 2007; Jegou et al., 2008; Mulder and Etienne, 2010). The appearance of these features correlates with a second abrupt decrease in slope gradient (from 0.9 to 0.3∘) and with the transition from bedded echofacies with low penetration to rough echofacies indicating a change in the sedimentary process and suggesting a high sand∕mud ratio. This area corresponds to deposition by spreading flows in an unchanneled area referred to as the intermediate depocenter in Fig. 13 and covering surface area of ca. 4250 km2. However, the low penetration of the 3.5 kHz echosounder and the limited number of seismic lines in this area did not allow a more detailed interpretation of the sedimentary processes in this part of the system.
5.1.3 Mid-system valley and distal lobe complexes
The presence of a steeper slope downslope of the intermediate depocenter (0.6∘) led to the incision of the multi-sourced mid-system valley, which acts as an outlet channel for turbidity currents that are energetic enough to travel through the flatter depositional area (Fig. 13b). The numerous erosional scars present in the upstream part of the valley suggest that this section has migrated upstream by retrogressive erosion, whereas the downstream part appears more stable with a straighter pathway and steeper flanks, these features being similar to the Tanzania Channel described by Bourget et al. (2008). According to the available bathymetric data, the volume of sediment removed from the mid-system valley is between 8 and 10 km3. The pathway of the valley seems to be controlled by the seafloor topography as the valley deviates near the rocky seamount located west of São Tomé. This large mid-system valley delivers sediments to the lower fan.
At the outlet of the mid-system valley, the echofacies shows an area mainly characterized by rough echofacies (III) forming stacked lenses. This organization is characteristic of sandy lobe deposits (Kenyon et al., 1995; Piper and Normark, 2001). This area, referred as the upper lobe area in Fig. 13, constitutes the main lobe complex (sensu Prélat and Hodgson, 2013) of the Ogooue Fan. Core KC11 shows that coarse-grained turbidity currents are deposited in the proximal part of the lobe complex. The abrupt transitions between erosional or bypass and depositional behavior observed notably at the mouth of the mid-system valley is the result of hydraulic jumps affecting flows when they become unconfined between channel sides and spread laterally (Komar, 1971; Garcia and Parker, 1989). According to the seismic data, the depositional area of the lobe complex is ∼100 km long, reaches ∼40 km in width, spreads over 2860 km2 and reaches up to 40 m in thickness. The transparent lenses are interpreted as lobes: they seem to be bounded by erosive bases and separated vertically by fine-grained units (Mulder and Etienne, 2010; Prélat and Hodgson, 2013). Some incisions (< 15 m deep) are imaged on the top surface of the lobes; two of them are visible in Fig. 12. The area where incisions are present is interpreted as the channelized part of the lobe complex. This lobe area presents a gentle slope (0.3∘) oriented north–south, suggesting that topographic compensation would shift future lobe deposition southward. However, the few numbers of seismic lines do not allow the precise internal geometry and the timing of the construction of the different lobe units.
This depositional area is not the distalmost part of the Ogooue Fan. West of this lobe, evidences of active sedimentation are visible on the reflectivity map (Figs. 2, 4). The reflectivity map shows high-backscatter finger-shape structures suggesting pathways of gravity flows (Fig. 2b, detail A). These lineations (< 10 m deep) are concentrated in a 20 km wide corridor just west of the lobe area and then form a wider area extending up to 550 km offshore the Ogooue Delta. This part of the system follows the same pattern as the one previously described between the intermediate depocenter and the upper lobe area (Fig. 13b). The corridor appears on a segment of steeper slope (0.3∘) just at the downslope end of the upper lobe area (0.2∘). This corridor, which disappears when the slope becomes gentler (0.1∘), is certainly dominated by sediment bypass (sensu Stevenson et al., 2015). Core KC15, located downstream of this corridor in the lower lobe area, is composed of very thin silty turbidites corresponding to the upper parts of the Bouma sequence interbedded with hemipelagic deposits. The upper lobe acts as a trap for the basal sand-rich parts of gravity flows, and the lower lobe area receives only the upper part of the flows, which is composed of fine-grained sediments. The spatial distribution of facies suggests a filling of successive depocenters with a downslope decrease in the coarse-grained sediment proportion (Fig. 6).
Considering the sedimentary facies of core KC15 located downstream this corridor, we can assume that this corridor was formed by the repeated spill-over of the fine-grained top of turbidity currents over the upper lobe area. This architecture suggests that this corridor is dominated by sediment bypass (sensu Stevenson et al., 2015). On the most distal segment with a very low slope gradient (0.1–0.2∘) sediment deposition dominates.
5.1.4 Isolated systems
On the northern part of the slope, the isolated system composed of the Cape Lopez Canyon, Cape Lopez intra-slope lobe, channel A and northern lobe follows the same pattern (Fig. 13c). The Cape Lopez intra-slope lobe occupies a small confined basin, 6 km wide and 16 km long and covers an area of 106 km2. This lobe appears to be very similar to the “X fan” described in Jobe et al. (2017) on the Niger Delta slope (8 km × 8 km, 76 km2) and is in the same size range as the intra-slope complexes studied in the Karoo Basin by Spychala et al. (2015) (6–10 km wide and 15–25 km). The two successive depositional areas, composed by the Cape Lopez lobe and the northern lobe, are located on areas with a low slope gradient (0.6–0.3∘) whereas erosion and sediment bypass dominate on segments of steeper slope gradient (1.6∘). The high slope gradient between the two depositional areas favored the construction of a straight deeply entrenched channel (> 100 m deep near the knickpoints) without levee (Fig. 7b) instead of a large valley similar to the central mid-system valley.
In the southern part of the fan, channel F transports sediments southward (Fig. 13d). At 2200 m water depth, a transparent echofacies appears associated with the pathway of this channel. This echofacies suggests that sediment transported by this channel might be partly deposited in this area by turbidity current overflow. This channel might also be associated with a depositional lobe; however, the area covered by the MOCOSED survey does not allow us to image it.
5.2 The Ogooue Fan among other complex slope fans
The Ogooue Fan develops on a stepped slope (Prather, 2003), which creates a succession of depositional areas on segments with gentle slope (referred to as “steps” in Smith, 2004) and segments of steeper slope (“ramps” in Smith, 2004) associated with erosion or sediment bypass (Fig. 13) (Demyttenaere et al., 2000; Deptuck et al., 2012; O'Byrne et al., 2004; Smith, 2004). The depositional behavior in these systems is guided by an equilibrium profile of the system that forms preferential areas of sedimentation or erosion (Komar, 1971; Ferry et al., 2005). As described in the conceptual model of O'Byrne et al. (2004), erosion is favored where local gradient increases, the eroded sediments being delivered downstream resulting in a local increase in sediment load (O'Byrne et al., 2004; Gee and Gawthorpe, 2006; Deptuck et al., 2012). This kind of fan geometry is common along the West African margin where abrupt changes in slope gradient and complex seafloor morphology are inherited from salt tectonic movement (Pirmez et al., 2000; Ferry et al., 2005; Gee and Gawthorpe, 2006; Gee et al., 2007). Deptuck et al. (2012) has described the influence of stepped slope on sedimentary processes along the western Niger Delta. They showed that differences in slope gradient between ramps (0.8 to 2.1∘) and steps (0.3 to 1.1∘) induce the transition from vertical incision and sediment removal to preferential sediment accumulation (Deptuck et al., 2007; Deptuck, 2012). Gradient changes along the Gabonese margin are however lower than the ones reported in Deptuck et al. (2012) and variation in slope gradient of 0.2∘ appears to be enough to modify sedimentary processes. The impact of subtle changes in slope gradients has already been highlighted by studies of the Karoo Basin (Van der Merwe et al., 2014; Spychala et al., 2015; Brooks et al., 2018) and Moroccan margin where sedimentary processes are controlled by very subtle gradient changes (< 0.1∘) (Stevenson et al., 2013; Wynn et al., 2012).
Moreover, in the modern Ogooue Fan, the presence of several bathymetric highs including the volcanic islands of the CVL and Mount Loiret acts as obstacles for the flows and creates a more complex slope profile. Such topographic highs are not present in the Congo and Niger systems. The bathymetric highs on the Ogooue fan area induce a lateral shift of the pathways of different channels as well as the pathway of the mid-system valley and form several downslope depositional lobes such as the Cape Lopez lobe that is constrained by the presence of Mount Loiret. Several complex-slope systems have already been described in the literature with slope complexity due to salt-related deformations, e.g., the Gulf of Mexico (Prather et al., 1998; Beaubouef and Friedmann, 2000), offshore Angola (Hay, 2012) or basin thrusting (offshore Brunei; McGilvery and Cook, 2003, Markan margin; Bourget et al., 2010). For these systems, the slope evolves rapidly, and sedimentation and erosion are unlikely to establish an equilibrium profile. In contrast, the Gabonese margin reached a mature evolutionary stage with salt diapir piercement rate much lower than the deposition rate and thus no conspicuous effect of salt tectonics on the deposition of overburden sediment (Chen et al., 2007). Sedimentation and erosion certainly dominate the short-term evolution of the slope. The Ogooue Fan appears to be much more similar to the morphology of the northwest African margin where the Madeira, the Canary and the Cabo Verde islands create a complex slope morphology along the Moroccan and Mauritanian margin (Masson, 1994; Wynn et al., 2000, 2002, 2012).
This study provides the first data on the morphology of the recent Ogooue deep-sea fan and interpretations on sedimentary processes occurring in this environment. The Gabonese margin presents a pelagic or hemipelagic background sedimentation overprinted by downslope gravity flows. The fan is made up of various architectural elements and consists of both constructional and erosional sections. The pattern of sedimentation on the margin is controlled by subtle slope gradient changes (< 0.3∘). The long-term interaction between gravity flows and the seafloor topography has induced the construction of successive depocenters and sediment bypass areas. The gravity flows have modified the topography according to a theoretical equilibrium profile, eroding the seafloor where slopes are steeper than the theoretical equilibrium profiles and depositing sediments when slopes are gentler than the theoretical equilibrium profile. Three successive main sediment depocenters have been identified along a longitudinal profile. They are associated with three areas of low slope gradient (0.3–0.2∘). The two updip deposition areas – the intermediate depocenter and the upper lobe area – have recorded coarse-grained sedimentation and are connected by a well-developed large mid-system valley measuring 100 km long and located on a steeper slope segment (0.6∘). The distalmost depocenter – the lower lobe area – receives only the fine-grained portion of the sediment load that has bypassed the more proximal deposit areas. Sedimentation on this margin is made more complex by the presence of several volcanic islands and seamounts that constrain the gravity flows. The presence on the slope of Mount Loiret has caused the formation of an isolated system composed of the Cape Lopez Canyon and lobe, which continues downstream by the Northern Lobe area.
All the raw data belong to the SHOM (hydrological and oceanographic marine service), which is a French military institution; it cannot, therefore, be placed in a public repository. The interpreted maps can be available on request to the main author.
SM, TM and PM performed the analysis. SM wrote the paper in consultation with TM and PM. TG collected the data and provided critical feedbacks on the project.
The authors declare that they have no conflict of interest.
We thank the SHOM (hydrological and oceanographic marine service) for the data, the ARTEMIS technical platform for radiocarbon age dating. We are also grateful to EPOC technicians and engineers – Isabelle Billy, Pascal Lebleu, Olivier Ther – for the data acquisition. Jacob Covault, Peter Haughton and David Mark Hodgson are thanked for their constructive and helpful reviews.
This paper was edited by Elias Samankassou and reviewed by David M. Hodgson, Peter Haughton, and Jacob Covault.
Amy, L. A., Kneller, B. C., and McCaffrey, W. D.: Facies architecture of the Grès de Peïra Cava, SE France: landward stacking patterns in ponded turbiditic basins, J. Geol. Soc., 164, 143–162, https://doi.org/10.1144/0016-76492005-019, 2007.
Anka, Z., Séranne, M., Lopez, M., Scheck-Wenderoth, M., and Savoye, B.: The long-term evolution of the Congo deep-sea fan: A basin-wide view of the interaction between a giant submarine fan and a mature passive margin (ZaiAngo project), Tectonophysics, 470, 42–56, https://doi.org/10.1016/j.tecto.2008.04.009, 2009.
Babonneau, N., Savoye, B., Cremer, M., and Klein, B.: Morphology and architecture of the present canyon and channel system of the Zaire deep-sea fan, Mar. Petrol. Geol., 19, 445–467, https://doi.org/10.1016/S0264-8172(02)00009-0, 2002.
Barfod, D. N. and Fitton, J. G.: Pleistocene volcanism on São Tomé, Gulf of Guinea, West Africa, Quat. Geochronol., 21, 77–89, https://doi.org/10.1016/j.quageo.2012.11.006, 2014.
Beaubouef, R. T. and Friedmann, S. J.: High resolution seismic/sequence stratigraphic framework for the evolution of Pleistocene intra slope basins, western Gulf of Mexico: depositional models and reservoir analogs, in: Deepwater Reservoirs of the World, Presented at the SEPM, 20th Annual Research Conference, 40–60, 2000.
Bertoni, C. and Cartwright, J.: 3D seismic analysis of slope-confined canyons from the Plio-Pleistocene of the Ebro Continental Margin (Western Mediterranean), Basin Res., 17, 43–62, https://doi.org/10.1111/j.1365-2117.2005.00254.x, 2005.
Biscara, L., Mulder, T., Martinez, P., Baudin, F., Etcheber, H., Jouanneau, J.-M., and Garlan, T.: Transport of terrestrial organic matter in the Ogooué deep sea turbidite system (Gabon), Mar. Petrol. Geol., 28, 1061–1072, https://doi.org/10.1016/j.marpetgeo.2010.12.002, 2011.
Biscara, L., Mulder, T., Hanquiez, V., Marieu, V., Crespin, J.-P., Braccini, E., and Garlan, T.: Morphological evolution of Cap Lopez Canyon (Gabon): Illustration of lateral migration processes of a submarine canyon, Mar. Geol., 340, 49–56, https://doi.org/10.1016/j.margeo.2013.04.014, 2013.
Bourget, J., Zaragosi, S., Garlan, T., Gabelotaud, I., Guyomard, P., Dennielou, B., Ellouz-Zimmermann, N., and Schneider, J.: Discovery of a giant deep-sea valley in the Indian Ocean, off eastern Africa: The Tanzania channel, Mar. Geol., 255, 179–185, https://doi.org/10.1016/j.margeo.2008.09.002, 2008.
Bourget, J., Zaragosi, S., Ellouz-Zimmermann, S., Ducassou, E., Prins, M. A., Garlan, T., Lanfumey, V., Schneider, J.-L., Rouillard, P., and Giraudeau, J.: Highstand vs. lowstand turbidite system growth in the Makran active margin: Imprints of high-frequency external controls on sediment delivery mechanisms to deep water systems, Mar. Geol., 274, 187–208, https://doi.org/10.1016/j.margeo.2010.04.005, 2010.
Bourgoin, J., Reyre, D., Magloire, P., and Krichewsky, M.: Les canyons sous-marins du cap Lopez (Gabon), Cah Ocean., 6, 372–387, 1963.
Brooks, H. L., Hodgson, D. M., Brunt, R. L., Peakall, J., Poyatos-Moré, M., and Flint, S. S.: Disconnected submarine lobes as a record of stepped slope evolution over multiple sea-level cycles, Geosphere, 14, 1753–1779, https://doi.org/10.1130/GES01618.1, 2018.
Cameron, N. R. and White, K.: Exploration Opportunities in Offshore Deepwater Africa, IBC Oil Gas Dev. West Afr. Lond., UK, 1999.
Chen, J.-C., Lo, C. Y., Lee, Y. T., Huang, S. W., Chou, P. C., Yu, H. S., Yang, T. F., Wang, Y. S., and Chung, S. H.: Mineralogy and chemistry of cored sediments from active margin off southwestern Taiwan, Geochem. J., 41, 303–321, 2007.
Cita, M. B., Beghi, C., Camerlenghi, A., Kastens, K. A., McCoy, F. W., Nosetto, A., Parisi, E., Scolari, F., and Tomadin, L.: Turbidites and megaturbidites from the Herodotus abyssal plain (eastern Mediterranean) unrelated to seismic events, Mar. Geol., 55, 79–101, https://doi.org/10.1016/0025-3227(84)90134-8, 1984.
Clift, P. and Gaedicke, C.: Accelerated mass flux to the Arabian Sea during the middle to late Miocene, Geology, 30, 207–210, https://doi.org/10.1130/0091-7613(2002)030<0207:AMFTTA>2.0.CO;2, 2002.
Covault, J. A., Romans, B. W., Fildani, A., McGann, M., and Graham, S. A.: Rapid Climatic Signal Propagation from Source to Sink in a Southern California Sediment-Routing System, J. Geol., 118, 247–259, https://doi.org/10.1086/651539, 2010.
Covault, J. A., Romans, B. W., Graham, S. A., Fildani, A., and Hilley, G. E.: Terrestrial source to deep-sea sink sediment budgets at high and low sea levels: Insights from tectonically active Southern California, Geology, 39, 619–622, https://doi.org/10.1130/G31801.1, 2011.
Covault, J. A., Shelef, E., Traer, M., Hubbard, S. M., Romans, B. W., and Fildani, A.: Deep-water channel run-out length: Insights from seafloor geomorphology, J. Sediment. Res., 82, 21–36, 2012.
Damuth, J.: The Amazon-HARP Fan Model: Facies Distributions in Mud-Rich Deep-Sea Fans Based on Systematic Coring of Architectural Elements of Amazon Fan, 1995.
Damuth, J. E.: Use of high-frequency (3.5–12 kHz) echograms in the study of near-bottom sedimentation processes in the deep-sea: a review, Mar. Geol., 38, 51–75, 1980a.
Damuth, J. E.: Quaternary sedimentation processes in the South China Basin as revealed by echo-character mapping and piston-core studies, in: Geophysical Monograph Series, edited by: Hayes, D. E., American Geophysical Union, Washington, D. C., 105–125, https://doi.org/10.1029/GM023p0105, 1980b.
Damuth, J. E.: Echo character of the western equatorial Atlantic floor and its relationship to the dispersal and distribution of terrigenous sediments, Mar. Geol., 18, 17–45, https://doi.org/10.1016/0025-3227(75)90047-X, 1975.
Damuth, J. E.: Neogene gravity tectonics and depositional processes on the deep Niger Delta continental margin, Mar. Petrol. Geol., 11, 320–346, https://doi.org/10.1016/0264-8172(94)90053-1, 1994.
Damuth, J. E. and Hayes, D. E.: Echo character of the East Brazilian continental margin and its relationship to sedimentary processes, Mar. Geol., 24, 73–95, https://doi.org/10.1016/0025-3227(77)90002-0, 1977.
Demyttenaere, R., Tromp, J. P., Ibrahim, A., and Allman-Ward, P.: Brunei Deep Water Exploration: From Sea Floor Images and Shallow Seismic Analogues to Depositional Models in a Slope Turbidite Setting, in: Deep-Water Reservoirs of the World: 20th Annual. Society of economic palaeontologists and mineralogists, edited by: Weimer, P., 304–317, https://doi.org/10.5724/gcs.00.20, 2000.
Deptuck, M. E.: Pleistocene Seascape Evolution Above A “Simple” Stepped Slope–Western Niger Delta, in: Application of the Principles of Seismic Geomorphology to Continental-Slope and Base-of-Slope Systems: Case Studies from Seafloor and Near-Seafloor Analogues, edited by: Prather, B. E., Deptuck, M. E., Mohrig, D., Van Hoorn, B., and Wynn, R. B., SEPM, https://doi.org/10.2110/pec.12.99, 2012.
Deptuck, M. E., Steffens, G. S., Barton, M., and Pirmez, C.: Architecture and evolution of upper fan channel-belts on the Niger Delta slope and in the Arabian Sea, Mar. Petrol. Geol., 20, 649–676, https://doi.org/10.1016/j.marpetgeo.2003.01.004, 2003.
Deptuck, M. E., Sylvester, Z., Pirmez, C., and O'Byrne, C.: Migration–aggradation history and 3-D seismic geomorphology of submarine channels in the Pleistocene Benin-major Canyon, western Niger Delta slope, Mar. Petrol. Geol., 24, 406–433, https://doi.org/10.1016/j.marpetgeo.2007.01.005, 2007.
Déruelle, B., Ngounouno, I., and Demaiffe, D.: The “Cameroon Hot Line” (CHL): A unique example of active alkaline intraplate structure in both oceanic and continental lithospheres, C. R. Geosci., 339, 589–600, https://doi.org/10.1016/j.crte.2007.07.007, 2007.
Dill, R. F., Dietz, R. S., and Stewart, H.: deep-sea channels and delta of the Monterey submarine canyon, Geol. Soc. Am. Bull., 65, 191–194, 1954.
Droz, L., Rigaut, F., Cochonat, P., and Tofani, R.: Morphology and recent evolution of the Zaire turbidite system (Gulf of Guinea), Geol. Soc. Am. Bull., 108, 253–269, https://doi.org/10.1130/0016-7606(1996)108<0253:MAREOT>2.3.CO;2, 1996.
Droz, L., Marsset, T., Ondras, H., Lopez, M., Savoye, B., and Spy-Anderson, F.-L.: Architecture of an active mud-rich turbidite system: The Zaire Fan (Congo–Angola margin southeast Atlantic): Results from ZaAngo 1 and 2 cruises, AAPG Bull., 87, 1145–1168, 2003.
Embley, R. W.: New evidence for occurrence of debris flow deposits in the deep sea, Geology, 4, 371–374, https://doi.org/10.1130/0091-7613(1976)4<371:NEFOOD>2.0.CO;2, 1976.
Ferry, J.-N., Mulder, T., Parize, O., and Raillard, S.: Concept of equilibrium profile in deep-water turbidite system: effects of local physiographic changes on the nature of sedimentary process and the geometries of deposits, Geol. Soc. Lond. Spec. Publ., 244, 181–193, https://doi.org/10.1144/GSL.SP.2005.244.01.11, 2005.
Field, M. E. and Gardner, J. V.: Pliocene-Pleistocene growth of the Rio Ebro margin, northeast Spain: A prograding-slope model, Geol. Soc. Am. Bull., 102, 721–733, https://doi.org/10.1130/0016-7606(1990)102<0721:PPGOTR>2.3.CO;2, 1990.
Fildani, A. and Normark, W. R.: Late Quaternary evolution of channel and lobe complexes of Monterey Fan, Mar. Geol., 206, 199–223, https://doi.org/10.1016/j.margeo.2004.03.001, 2004.
Garcia, M. and Parker, G.: Experiments on hydraulic jumps in turbidity currents near a canyon-fan transition, Science, 245, 393–396, https://doi.org/10.1126/science.245.4916.393, 1989.
Garlan, T., Biscara, L., Guyomard, P., Le Faou, Y., and Gabelotaud, I.: Rapport de la campagne MOCOSED 2010, Modèle de couches sédimentaires du Golfe de Guinée (Rapport de mission), SHOM, 2010.
Gaullier, V. and Bellaiche, G.: Near-bottom sedimentation processes revealed by echo-character mapping studies, north-western Mediterranean Basin, AAPG Bull., 82, 1140–1155, 1998.
Gay, A., Lopez, M., Cochonat, P., Sultan, N., Cauquil, E., and Brigaud, F.: Sinuous pockmark belt as indicator of a shallow buried turbiditic channel on the lower slope of the Congo basin, West African margin, Geol. Soc. Lond. Spec. Publ., 216, 173–189, https://doi.org/10.1144/GSL.SP.2003.216.01.12, 2003.
Gee, M. J. R. and Gawthorpe, R. L.: Submarine channels controlled by salt tectonics: Examples from 3D seismic data offshore Angola, Mar. Petrol. Geol., 23, 443–458, https://doi.org/10.1016/j.marpetgeo.2006.01.002, 2006.
Gee, M. J. R., Gawthorpe, R. L., Bakke, K., and Friedmann, S. J.: Seismic Geomorphology and Evolution of Submarine Channels from the Angolan Continental Margin, J. Sediment. Res., 77, 433–446, https://doi.org/10.2110/jsr.2007.042, 2007.
Giresse, P.: Carte sédimentologique des fonds sous-marins du delta de l'Ogooué, Cahiers Océanographiques, 10, 965–994, 1969.
Giresse, P. and Odin, G. S.: Nature minéralogique et origine des glauconies du plateau continental du Gabon et du Congo, Sedimentology, 20, 457–488, 1973.
Guillou, R.: MOCOSED 2010 cruise, Pourquoi pas??, https://doi.org/10.17600/10030110, 2010.
Hanquiez, V., Mulder, T., Lecroart, P., Gonthier, E., Marchès, E., and Voisset, M.: High resolution seafloor images in the Gulf of Cadiz, Iberian margin, Mar. Geol., 246, 42–59, https://doi.org/10.1016/j.margeo.2007.08.002, 2007.
Hay, D.: Stratigraphic evolution of a tortuous corridor from the stepped slope of Angola, in: Application of the Principles of Seismic Geomorphology to Continental-Slope and Base-of-Slope Systems: Case Studies from Seafloor and Near-Seafloor Analogues, edited by: Prather, B. E., Deptuck, M. E., Mohrig, D., Van Hoorn, B., and Wynn, R. B., SEPM Spec. P., 99, 163–180, https://doi.org/10.2110/pec.12.99, 2012.
Heezen, B. C., Tharp, M., and Ewing, M.: The Floors of the Oceans, in: Geological Society of America Special Papers, Geol. Soc. Am. S., 65, 1–126, https://doi.org/10.1130/SPE65-p1, 1959.
Jacobi, R. D.: Sediment slides on the northwestern continental margin of Africa, Mar. Geol., 22, 157–173, https://doi.org/10.1016/0025-3227(76)90045-1, 1976.
Jegou, I., Savoye, B., Pirmez, C., and Droz, L.: Channel-mouth lobe complex of the recent Amazon Fan: The missing piece, Mar. Geol., 252, 62–77, https://doi.org/10.1016/j.margeo.2008.03.004, 2008.
Jobe, Z. R., Lowe, D. R., and Uchytil, S. J.: Two fundamentally different types of submarine canyons along the continental margin of Equatorial Guinea, Mar. Petrol. Geol., 28, 843–860, https://doi.org/10.1016/j.marpetgeo.2010.07.012, 2011.
Jobe, Z. R., Sylvester, Z., Howes, N., Pirmez, C., Parker, A., Cantelli, A., Smith, R., Wolinsky, M. A., O'Byrne, C., Slowey, N., and Prather, B.: High-resolution, millennial-scale patterns of bed compensation on a sand-rich intraslope submarine fan, western Niger Delta slope, Geol. Soc. Am. Bull., 129, 23–37, https://doi.org/10.1130/B31440.1, 2017.
Kane, I. A., Catterall, V., McCaffrey, W. D., and Martinsen, O. J.: Submarine channel response to intrabasinal tectonics: The influence of lateral tilt, AAPG Bull., 94, 189–219, https://doi.org/10.1306/08180909059, 2010.
Kenyon, N. H., Millington, J., Droz, L., and Ivanov, M. K.: Scour holes in a channel-lobe transition zone on the Rhône Cone, in: Atlas of Deep Water Environments, Springer, Dordrecht, 212–215, https://doi.org/10.1007/978-94-011-1234-5_31, 1995.
Kneller, B.: Beyond the turbidite paradigm: physical models for deposition of turbidites and their implications for reservoir prediction, Geol. Soc. Lond. Spec. Publ., 94, 31–49, https://doi.org/10.1144/GSL.SP.1995.094.01.04, 1995.
Kolla, V.: A review of sinuous channel avulsion patterns in some major deep-sea fans and factors controlling them, Mar. Petrol. Geol., 24, 450–469, https://doi.org/10.1016/j.marpetgeo.2007.01.004, 2007.
Komar, P. D.: Hydraulic jumps in turbidity currents, Bull. Geol. Soc. Am., 82, 1477–1488, 1971.
Lebigre, J. M.: Les mangroves des rias du littoral gabonais, essai de cartographie typologique, Rev. Bois For. Trop., 1983.
Lee, D.-C., Halliday, A. N., Fitton, J. G., and Poli, G.: Isotopic variations with distance and time in the volcanic islands of the Cameroon line: evidence for a mantle plume origin, Earth Planet. Sc. Lett., 123, 119–138, https://doi.org/10.1016/0012-821X(94)90262-3, 1994.
Lerique, J., Barret, J., and Walter, R.: Hydrographie, hydrologie, in: Géographie et cartographie du Gabon: atlas illustré, EDICEF, Paris, 14–17, 1983.
Loncke, L., Droz, L., Gaullier, V., Basile, C., Patriat, M., and Roest, W.: Slope instabilities from echo-character mapping along the French Guiana transform margin and Demerara abyssal plain, Mar. Petrol. Geol., 26, 711–723, https://doi.org/10.1016/j.marpetgeo.2008.02.010, 2009.
Lonergan, L., Jamin, N. H., Jackson, C. A.-L., and Johnson, H. D.: U-shaped slope gully systems and sediment waves on the passive margin of Gabon (West Africa), Mar. Geol., 337, 80–97, https://doi.org/10.1016/j.margeo.2013.02.001, 2013.
Mahé, G., Lerique, J., and Olivry, J.-C.: Le fleuve Ogooué au Gabon: reconstitution des débits manquants et mise en évidence de variations climatiques à l'équateur, Hydrol. Cont., 5, 105–124, 1990.
Masson, D. G.: Late Quaternary turbidity current pathways to the Madeira Abyssal Plain and some constraints on turbidity current mechanisms, Basin Res., 6, 17–33, https://doi.org/10.1111/j.1365-2117.1994.tb00072.x, 1994.
Masson, D. G., Kenyon, N. H., Gardner, J. V., and Field, M. E.: Monterey Fan: channel and overbank morphology, in: Atlas of Deep Water Environments, edited by: Pickering, K. T., Hiscott, R. N., Kenyon, N. H., Ricci Lucchi, F., and Smith, R. D. A., Springer Netherlands, Dordrecht, 74–79, https://doi.org/10.1007/978-94-011-1234-5_13, 1995.
McGilvery, T. A. and Cook, D. L.: The Influence of Local Gradients on Accommodation Space and Linked Depositional Elements Across a Stepped Slope Profile, Offshore Brunei, in: Shelf Margin Deltas and Linked Down Slope Petroleum Systems: 23rd Annual, edited by: Roberts, H. R., Rosen, N. C., Fillon, R. F., and Anderson, J. B., Society of Economic Paleontologists and Mineralogists, https://doi.org/10.5724/gcs.03.23, 2003.
Menard, H. W.: Deep-Sea Channels, Topography, and Sedimentation, AAPG Bull., 39, 236–255, 1955.
Migeon, S., Weber, O., Faugeres, J.-C., and Saint-Paul, J.: SCOPIX: A new X-ray imaging system for core analysis, Geo-Mar. Lett. 18, 251–255, https://doi.org/10.1007/s003670050076, 1998.
Mignard, S. L.-A., Mulder, T., Martinez, P., Charlier, K., Rossignol, L., and Garlan, T.: Deep-sea terrigenous organic carbon transfer and accumulation: Impact of sea-level variations and sedimentation processes off the Ogooue River (Gabon), Mar. Petrol. Geol., 85, 35–53, https://doi.org/10.1016/j.marpetgeo.2017.04.009, 2017.
Mougamba, R.: Chronologie et architechture des systems turbiditiquess Cénozoïques du Prisme sédimentaire de l'Ogooué (Marge Nord-Gabon), Université de Lille, Lille, 1999.
Mouscardes, P.: OPTIC CONGO 2 cruise, RV Beautemps-Beaupré, available at: https://campagnes.flotteoceanographique.fr/campagnes/5090050/fr/ (last access: 7 May 2018), 2005.
Mulder, T. and Alexander, J.: Abrupt change in slope causes variation in the deposit thickness of concentrated particle-driven density currents, Mar. Geol., 175, 221–235, https://doi.org/10.1016/S0025-3227(01)00114-1, 2001.
Mulder, T. and Etienne, S.: Lobes in deep-sea turbidite systems: State of the art, Sediment. Geol., 229, 75–80, https://doi.org/10.1016/j.sedgeo.2010.06.011, 2010.
Normark, W. R. and Damuth, J. E.: Sedimentary facies and associated depositional elements of the Amazon Fan, Proceedings of the Ocean Drilling Program, Ocean Drilling Program, https://doi.org/10.2973/odp.proc.sr.155.1997, 1997.
Normark, W. R. and Piper, D. J. W.: Initiation processes and flow evolution of turbidity currents: implications for the depositional record, in: From Shoreline to Abyss, SEPM Spec. P., 207–230, 1991.
Normark, W. R., Barnes, N. E., and Coumes, F.: Rhone Deep-Sea Fan: A review, Geo-Mar. Lett., 3, 155–160, https://doi.org/10.1007/BF02462461, 1983.
Normark, W. R., Posamentier, H., and Mutti, E.: Turbidite systems: State of the art and future directions, Rev. Geophys., 31, 91–116, https://doi.org/10.1029/93RG02832, 1993.
O'Byrne, C., Prather, B., Pirmez, C., and Steffens, G. S.: Reservoir architectural styles across stepped slope profiles: Implications for exploration, appraisal and development, Presented at the AAPG International conference, 24–27 October 2004, Cancun, Mexico, 2004.
Peakall, J., McCaffrey, B., and Kneller, B.: A Process Model for the Evolution, Morphology, and Architecture of Sinuous Submarine Channels, J. Sediment. Res., 70, 434–448, https://doi.org/10.1306/2DC4091C-0E47-11D7-8643000102C1865D, 2000.
Pettingill, H. S. and Weimer, P.: Worlwide deepwater exploration and production: Past, present, and future, Lead. Edge, 21, 371–376, https://doi.org/10.1190/1.1471600, 2002.
Pickering, K., Stow, D., Watson, M., and Hiscott, R.: Deep-water facies, processes and models: a review and classification scheme for modern and ancient sediments, Earth Sci. Rev., 23, 75–174, https://doi.org/10.1016/0012-8252(86)90001-2, 1986.
Pilcher, R. and Argent, J.: Mega-pockmarks and linear pockmark trains on the West African continental margin, Mar. Geol., 244, 15–32, https://doi.org/10.1016/j.margeo.2007.05.002, 2007.
Piper, D. J. W. and Normark, W. R.: Sandy fans-from Amazon to Hueneme and beyond, AAPG Bull., 85, 1407–1438, 2001.
Piper, D. J. W. and Normark, W. R.: Processes That Initiate Turbidity Currents and Their Influence on Turbidites: A Marine Geology Perspective, J. Sediment. Res., 79, 347–362, https://doi.org/10.2110/jsr.2009.046, 2009.
Pirmez, C., Beaubouef, R. T., Friedmann, S. J., and Mohrig, D. C.: Equilibrium Profile and Baselevel in Submarine Channels: Examples from Late Pleistocene Systems and Implications for the Architecture of Deepwater Reservoirs, in: Deep-Water Reservoirs of the World, edited by: Weimer, P., https://doi.org/10.5724/gcs.00.20, 2000.
Prather, B. E.: Controls on reservoir distribution, architecture and stratigraphic trapping in slope settings, Mar. Petrol. Geol., 20, 529–545, https://doi.org/10.1016/j.marpetgeo.2003.03.009, 2003.
Prather, B. E., Booth, J. R., Steffens, G. S., and Craig, P. A.: Classification, Lithologic Calibration, and Stratigraphic Succession of Seismic Facies of Intraslope Basins, Deep-Water Gulf of Mexico, AAPG Bull., 82, 701–728, 1998.
Prather, B. E., O'Byrne, C., Pirmez, C., and Sylvester, Z.: Sediment partitioning, continental slopes and base-of-slope systems, Basin Res., 29, 394–416, https://doi.org/10.1111/bre.12190, 2017.
Pratson, L. F. and Coakley, B. J.: A model for the headward erosion of submarine canyons induced by downslope-eroding sediment flows, Geol. Soc. Am. Bull., 108, 225–234, https://doi.org/10.1130/0016-7606(1996)108<0225:AMFTHE>2.3.CO;2, 1996.
Pratson, L. F. and Laine, E. P.: The relative importance of gravity-induced versus current-controlled sedimentation during the Quaternary along the Mideast U.S. outer continental margin revealed by 3.5 kHz echo character, Mar. Geol., 89, 87–126, https://doi.org/10.1016/0025-3227(89)90029-7, 1989.
Pratson, L. F., Ryan, W. B. F., Mountain, G. S., and Twichell, D. C.: Submarine canyon initiation by downslope-eroding sediment flows: Evidence in late Cenozoic strata on the New Jersey continental slope, Geol. Soc. Am. Bull., 106, 395–412, https://doi.org/10.1130/0016-7606(1994)106<0395:SCIBDE>2.3.CO;2, 1994.
Prélat, A. and Hodgson, D. M.: The full range of turbidite bed thickness patterns in submarine lobes: controls and implications, J. Geol. Soc., 170, 209–214, https://doi.org/10.1144/jgs2012-056, 2013.
Rasmussen, E. S.: Structural evolution and sequence formation offshore South Gabon during the Tertiary, Tectonophysics, 266, 509–523, https://doi.org/10.1016/S0040-1951(96)00236-3, 1996.
Reading, H. G. and Richards, M.: Turbidite systems in deep-water basin margins classified by grain size and feeder system, AAPG Bull., 78, 792–822, 1994.
Salles, L., Ford, M., and Joseph, P.: Characteristics of axially-sourced turbidite sedimentation on an active wedge-top basin (Annot Sandstone, SE France), Mar. Petrol. Geol., 56, 305–323, https://doi.org/10.1016/j.marpetgeo.2014.01.020, 2014.
Séranne, M. and Anka, Z.: South Atlantic continental margins of Africa: A comparison of the tectonic vs climate interplay on the evolution of equatorial West Africa and SW Africa margins, J. Afr. Earth Sci., 43, 283–300, https://doi.org/10.1016/j.jafrearsci.2005.07.010, 2005.
Séranne, M. and Nzé Abeigne, C.-R.: Oligocene to Holocene sediment drifts and bottom currents on the slope of Gabon continental margin (West Africa), Sediment. Geol., 128, 179–199, https://doi.org/10.1016/S0037-0738(99)00069-X, 1999.
Séranne, M., Bruguier, O., and Moussavou, M.: U-Pb single zircon grain dating of Present fluvial and Cenozoic aeolian sediments from Gabon: consequences on sediment provenance, reworking, and erosion processes on the equatorial West African margin, Bull. Société Géologique Fr., 179, 29–40, 2008.
Shepard, F. P.: submarine erosion, a discussion of recent papers, Geol. Soc. Am. Bull., 62, 1413, 1951.
Shepard, F. P.: Submarine Canyons: Multiple Causes and Long-Time Persistence, AAPG Bull., 65, 1062–1077, https://doi.org/10.1306/03B59459-16D1-11D7-8645000102C1865D, 1981.
Shepard, F. P. and Emery, K. O.: Submarine Topography off the California Coast: Canyons and Tectonic Interpretation, Geol. S. Am. S., 31, https://doi.org/10.1130/SPE31, 1941.
Smith, R.: Silled sub-basins to connected tortuous corridors: sediment distribution systems on topographically complex sub-aqueous slopes, Geol. Soc. Lond. Spec. Publ., 222, 23–43, https://doi.org/10.1144/GSL.SP.2004.222.01.03, 2004.
Spychala, Y. T., Hodgson, D. M., Flint, S. S., and Mountney, N. P.: Constraining the sedimentology and stratigraphy of submarine intraslope lobe deposits using exhumed examples from the Karoo Basin, South Africa, Sediment. Geol., 322, 67–81, https://doi.org/10.1016/j.sedgeo.2015.03.013, 2015.
Stanley, D. J. and Moore, G. T.: The Shelfbreak: Critical Interface on Continental Margins, SEPM, 33, https://doi.org/10.2110/pec.83.33, 1983.
Stevenson, C. J., Talling, P. J., Wynn, R. B., Masson, D. G., Hunt, J. E., Frenz, M., Akhmetzhanhov, A., and Cronin, B. T.: The flows that left no trace: Very large-volume turbidity currents that bypassed sediment through submarine channels without eroding the sea floor, Mar. Petrol. Geol., 41, 186–205, https://doi.org/10.1016/j.marpetgeo.2012.02.008, 2013.
Stevenson, C. J., Jackson, C. A.-L., Hodgson, D. M., Hubbard, S. M., and Eggenhuisen, J. T.: Deep-Water Sediment Bypass, J. Sediment. Res., 85, 1058–1081, https://doi.org/10.2110/jsr.2015.63, 2015.
Stow, D. A. V. and Piper, D. J. W.: Deep-water fine-grained sediments: facies models, Geol. Soc. Lond. Spec. Publ., 15, 611–646, https://doi.org/10.1144/GSL.SP.1984.015.01.38, 1984.
Sylvester, Z., Cantelli, A., and Pirmez, C.: Stratigraphic evolution of intraslope minibasins: Insights from surface-based model, AAPG Bull., 99, 1099–1129, https://doi.org/10.1306/01081514082, 2015.
Syvitski, J. P. M., Vörösmarty, C. J., Kettner, A. J., and Green, P.: Impact of Humans on the Flux of Terrestrial Sediment to the Global Coastal Ocean, Science, 308, 376–380, https://doi.org/10.1126/science.1109454, 2005.
Thornton, S. E.: Hemipelagites and Associated Facies of Slopes and Slope Basins, Geol. Soc. Lond. Spec. Publ., 15, 377–394, 1984.
Tripsanas, E. K., Phaneuf, B. A., and Bryant, W. R.: Slope Instability Processes in a Complex Deepwater Environment, Bryant Canyon Area, Northwest Gulf of Mexico, in: Offshore Technology Conference, Presented at the Offshore Technology Conference, Offshore Technology Conference, Houston, Texas, https://doi.org/10.4043/14273-MS, 2002.
Twichell, D. C. and Roberts, D. G.: Morphology, distribution, and development of submarine canyons on the United States Atlantic continental slope between Hudson arid Baltimore Canyons, Geology 10, 408–412, https://doi.org/10.1130/0091-7613(1982)10<408:MDADOS>2.0.CO;2, 1982.
Unterseh, S.: Cartographie et caractérisation du fond marin par sondeur multifaisceaux, Vandoeuvre-les-Nancy, INPL, 1999.
Van der Merwe, W. C., Hodgson, D. M., Brunt, R. L., and Flint, S. S.: Depositional architecture of sand-attached and sand-detached channel-lobe transition zones on an exhumed stepped slope mapped over a 2500 km2 area, Geosphere, 10, 1076–1093, https://doi.org/10.1130/GES01035.1, 2014.
Weaver, P. P. E., Wynn, R. B., Kenyon, N. H., and Evans, J.: Continental margin sedimentation, with special reference to the north-east Atlantic margin: Continental slope sedimentation, Sedimentology, 47, 239–256, https://doi.org/10.1046/j.1365-3091.2000.0470s1239.x, 2000.
Wonham, J., Jayr, S., Mougamba, R., and Chuilon, P.: 3D sedimentary evolution of a canyon fill (Lower Miocene-age) from the Mandorove Formation, offshore Gabon, Mar. Petrol. Geol., 17, 175–197, https://doi.org/10.1016/S0264-8172(99)00033-1, 2000.
Wynn, R. B., Masson, D. G., Stow, D. A., and Weaver, P. P.: Turbidity current sediment waves on the submarine slopes of the western Canary Islands, Mar. Geol., 163, 185–198, 2000.
Wynn, R. B., Weaver, P. P. E., Masson, D. G., and Stow, D. A. V.: Turbidite depositional architecture across three interconnected deep-water basins on the north-west African margin, Sedimentology, 49, 669–695, https://doi.org/10.1046/j.1365-3091.2002.00471.x, 2002.
Wynn, R. B., Cronin, B. T., and Peakall, J.: Sinuous deep-water channels: Genesis, geometry and architecture, Mar. Petrol. Geol., 24, 341–387, https://doi.org/10.1016/j.marpetgeo.2007.06.001, 2007.
Wynn, R. B., Talling, P. J., Masson, D. G., Le Bas, T. P., Cronin, B. T., and Stevenson, C. J.: The Influence of Subtle Gradient Changes on Deep-Water Gravity Flows: A Case Study From the Moroccan Turbidite System, in: Application of the Principles of Seismic Geomorphology to Continental-Slope and Base-of-Slope Systems: Case Studies from Seafloor and Near-Seafloor Analogues, edited by: Prather, B. E., Deptuck, M. E., Mohrig, D., Van Hoorn, B., and Wynn, R. B., SEPM, https://doi.org/10.2110/pec.12.99, 2012.