the Creative Commons Attribution 4.0 License.
the Creative Commons Attribution 4.0 License.
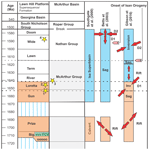
Basin inversion and structural architecture as constraints on fluid flow and Pb–Zn mineralization in the Paleo–Mesoproterozoic sedimentary sequences of northern Australia
George M. Gibson
Sally Edwards
As host to several world-class sediment-hosted Pb–Zn deposits and unknown quantities of conventional and unconventional gas, the variably inverted 1730–1640 Ma Calvert and 1640–1575 Ma Isa superbasins of northern Australia have been the subject of numerous seismic reflection studies with a view to better understanding basin architecture and fluid migration pathways. These studies reveal a structural architecture common to inverted sedimentary basins the world over, including much younger examples known to be prospective for oil and gas in the North Sea and elsewhere, with which they might be usefully compared. Such comparisons lend themselves to suggestions that the mineral and petroleum systems in Paleo–Mesoproterozoic northern Australia may have spatially, if not temporally overlapped and shared a common tectonic driver, consistent with the observation that basinal sequences hosting Pb–Zn mineralization in northern Australia are bituminous or abnormally enriched in hydrocarbons. Sediment-hosted Pb–Zn mineralization coeval with basin inversion first occurred during the 1650–1640 Ma Riversleigh Tectonic Event towards the close of the Calvert Superbasin with further pulses taking place during and subsequent to the onset of the 1620–1580 Ma Isa Orogeny and final closure of the Isa Superbasin. Mineralization is typically hosted by the post-rift or syn-inversion fraction of basin fill, contrary to existing interpretations of Pb–Zn ore genesis where the ore-forming fluids are introduced during the rifting or syn-extensional phase of basin development. Mineralizing fluids were instead expelled upwards during times of crustal shortening into structural and/or chemical traps developing in the hangingwalls of inverted normal faults. Inverted normal faults predominantly strike NNW and ENE, giving rise to a complex architecture of compartmentalized sub-basins whose individual uplifted basement blocks and doubly plunging periclinal folds exerted a strong control not only on the distribution and preservation of potential trap rocks but the direction of fluid flow, culminating in the co-location and trapping of mineralizing and hydrocarbon fluids in the same carbonaceous rocks. An important case study is the 1575 Ma Century Pb–Zn deposit where the carbonaceous host rocks served as both a reductant and basin seal during the influx of more oxidized mineralizing fluids, forcing the latter to give up their Pb and Zn metal. A transpressive tectonic regime in which basin inversion and mineralization were paired to folding, uplift, and erosion during arc–continent or continent–continent collision, and accompanied by orogen-parallel extensional collapse and strike-slip faulting best accounts for the observed relationships.
- Article
(23002 KB) - Full-text XML
- BibTeX
- EndNote
Northern Australia and its late-Paleoproterozoic–early-Mesoproterozoic basinal sequences have long attracted the interest of the minerals and petroleum exploration industries. Besides being the world's single largest repository of sediment-hosted Pb–Zn mineral deposits (Huston et al., 2006; Southgate et al., 2006), these same mineral-rich sequences hold some of the planet's oldest oil (Jackson et al., 1986) along with an unknown quantity of conventional and unconventional gas (Carr et al., 2019; Gorton and Troup, 2018; McConachie et al., 1993). Unsurprisingly, many mineral deposits and their host rocks are bituminous or contain very high proportions of organic carbon (Andrews, 1998; Broadbent et al., 1998; Hutton and Sweet, 1982; Jarrett et al., 2018; McConachie et al., 1993; McGoldrick et al., 2010), raising the possibility that the petroleum and mineralizing systems in northern Australia may have spatially, if not temporally, overlapped and share a common tectonic driver. Such a possibility was first entertained for the ca. 1575 Ma Century Pb–Zn deposit (Fig. 1a) where first hydrocarbons and then a more metalliferous ore-forming fluid are thought to have been sequentially trapped following their expulsion from deeper stratigraphic levels during folding and thrusting accompanying the 1620–1580 Ma Isan Orogeny (Broadbent et al., 1998). In this scenario, basin inversion was not only intimately linked to fluid migration and mineralization but played a key role in generating the structural architecture that brought the petroleum and mineralizing systems together in one place. Seismic reflection images for the Lawn Hill Platform have since shown the Century deposit to be hosted by the syn-inversion fraction of basin fill (Gibson et al., 2017, 2016) and to occur in rocks possessing a structural architecture common to inverted basins the world over, including those currently under exploration for oil and gas in the Irish and North seas and north European continental shelf more generally (Cooper et al., 1984; Hayward and Graham, 1989; Lowell, 1995; Thomas and Coward, 1995; Turner and Williams, 2004). Thus, not only does basin inversion appear to have been a prerequisite for ore formation at Century, but the structural architecture cannot have appreciably changed during the transition from a hydrocarbon to mineral system lest the similarities with their more modern European counterparts have been lost during crustal shortening. Such conclusions are difficult to reconcile with most existing models for sediment-hosted Pb–Zn mineralization in northern Australia where ore formation is interpreted to have been syn-extensional and facilitated by fluid migration along normal faults active at the time of basin formation (Huston et al., 2006; Large et al., 2005; Leach et al., 2010; McGoldrick et al., 2010). Alternative exploration strategies for this and other types of sediment-hosted Pb–Zn mineralization in northern Australia may therefore be warranted that better reflect the similarities with the petroleum system and target the structures formed during basin inversion. Here, we make use of publicly available industry and government deep seismic reflection data to show that inversion-related structures of more than one generation and style are widely developed in the late-Paleoproterozoic–early-Mesoproterozoic basin sequences of northern Australia (Figs. 1 and 2), reflecting successive episodes of crustal shortening during the course of which the majority of Pb–Zn deposits were emplaced (Gibson et al., 2017).
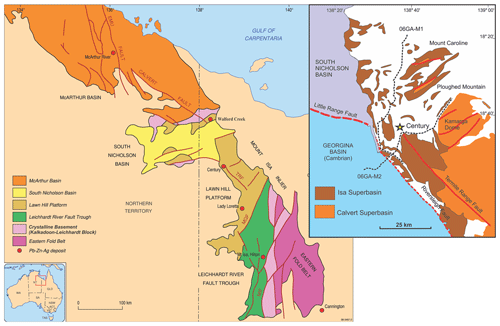
Figure 1(a) Simplified geological map for northern Australia showing principal tectono-morphological elements and Pb–Zn mineral deposits (after Jackson et al., 2000). (b) More detailed geological map of periclinal folds developed in stratigraphy of the inverted Isa Superbasin on the Lawn Hill Platform northeast of Century Mine and seismic reflection lines 06GAM1 and 06GAM2 along which these structures are imaged. Figure reproduced with permission under the Creative Commons Attribution 4.0 International License: http://creativecommons.org/licenses/by/4.0/legalcode (last access: 2 July 2020). © Commonwealth of Australia (Geoscience Australia) 2020.
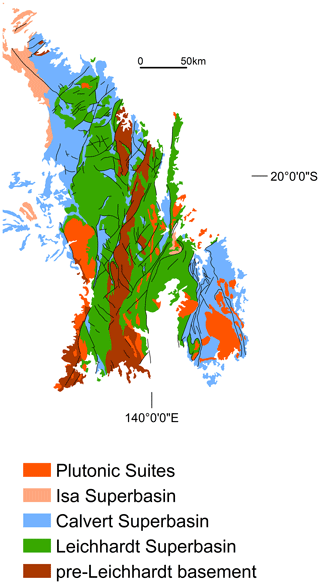
Figure 2Map showing presently defined limits of outcropping Leichhardt, Calvert, and Isa superbasins across the Mount Isa region. Seismic reflection data indicate that all three basins are variably preserved in the subsurface geology beneath the South Nicholson and Georgina basins and continue northwards into the McArthur Basin and Batten Trough (see Carr et al., 2019). Reproduced with permission: Licensed CC BY version 4.0. © State of Queensland, 2020.
Northern Australia's late-Paleoproterozoic–early-Mesoproterozoic basinal sequences belong to one of three superbasins (Figs. 2 and 3) which, together with the overlying Mesoproterozoic South Nicholson Basin (Fig. 1a), preserve a 500 million year history of lithospheric extension interrupted by successive episodes of basin inversion, uplift, and erosion (Betts et al., 2016; Gibson et al., 2012; Giles et al., 2002; Jackson et al., 2000; Neumann et al., 2006; Southgate et al., 2000a; Sweet, 2017; Yang et al., 2020). The oldest basin inversion event (Fig. 3) occurred after 1840 Ma and is best expressed by the angular unconformity separating the 6–8 km thick 1790–1740 Ma Leichhardt Superbasin (Fig. 2) from an older underlying ≥1870 Ma crystalline basement (Kalkadoon–Leichhardt Block; Fig. 2) variably intruded by foliated 1860–1840 Ma granites (Blake, 1987; Withnall and Hutton, 2013). Clasts of strongly foliated granite and other basement rocks occur widely in conglomerates at the base of the Leichhardt Superbasin (1790 Ma Bottletree Formation), but otherwise its basin fill is only mildly deformed and mainly comprises weakly metamorphosed (greenschist facies) continental tholeiites and rhyolite interstratified with subordinate but still substantial volumes of fluviatile to shallow-marine sedimentary rocks. This same cover–basement relationship is also evident on the Murphy Ridge (Fig. 4) farther north where conglomerates (Westmoreland Conglomerate) and sandstones (Wire Creek Sandstone) at the base of the 1790–1710 Ma Tawallah Group (Fig. 3) in the McArthur Basin (Fig. 1b) similarly rest unconformably on an older deformed basement intruded by 1860–1840 Ma granites (Ahmad and Munson, 2013; Rawlings et al., 2008; Sweet, 1984). As with the Kalkadoon–Leichhardt Block, basement granites on the Murphy Ridge were deformed long before the overlying conglomerate was deposited and likely represent exposed fragments of a much more regionally extensive magmatic belt that is continuous at depth and once lay at or close to the eastern margin of the North Australian Craton (Gibson et al., 2008; Korsch et al., 2012). Granites with calc-alkaline compositions occur widely throughout the Kalkadoon–Leichhardt Block (Bierlein et al., 2011) and may originally have formed part of a continental magmatic arc linked to west-dipping subduction beneath the eastern margin of the craton (Bierlein et al., 2008; Korsch et al., 2012). Alternatively, these granites originated in a backarc setting linked to oceanward retreat of a more distal arc built along either the southern or eastern margin of conjoined North and South Australian cratons (Betts et al., 2016; Betts and Giles, 2006; Gibson et al., 2018, 2012; Giles et al., 2002, 2004). Regardless of which interpretation is correct, by 1790 Ma lithospheric extension and thinning were well underway, and northern Australia was subjected to widespread intracontinental rifting, normal faulting, and half-graben formation accompanied at deeper crustal levels by elevated heat flow, low-pressure–high-temperature metamorphism, and bimodal magmatic intrusion (Betts et al., 2006; Gibson et al., 2012, 2008; Holcombe et al., 1991; O'Dea et al., 1997a; Pearson et al., 1991). Lithospheric extension during this phase of basin formation produced mainly northwest-oriented normal faults and half-graben and continued through until ca. 1740 Ma when backarc extension and rifting in the Mount Isa region and neighbouring McArthur Basin (Fig. 1a) temporarily ceased and gave way to an episode of thermal subsidence accompanied by the deposition of shallow marine quartzite and carbonate rocks (Gibson et al., 2012; Jackson et al., 2000; O'Dea et al., 1997b).
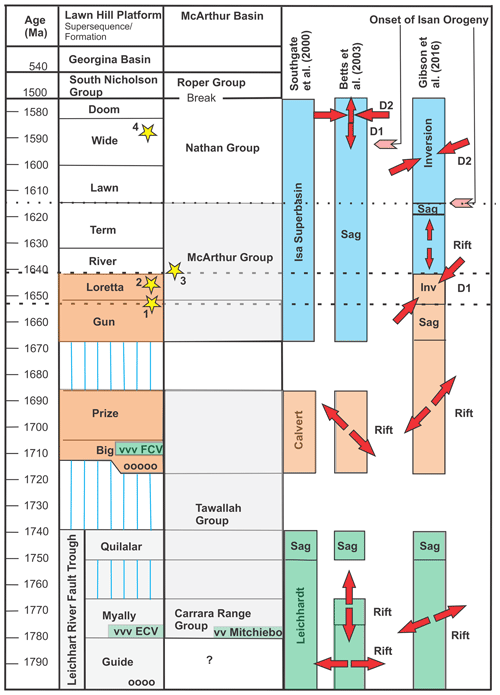
Figure 3Simplified stratigraphic column for Mount Isa region and neighbouring southern McArthur Basin showing three-fold subdivision into the Leichhardt, Calvert, and Isa superbasins but different interpretations of basin history and tectonic evolution (Betts and Lister, 2001; Betts et al., 2003; Gibson et al., 2016; Southgate et al., 2000a). The Carrara Range Group is shown as part of the lower Tawallah Group and a correlative of the Leichhardt Superbasin based on revised geological mapping (Rawlings et al., 2008) and recently published geochronological data (Kositcin and Carson, 2019) for the McArthur Basin. Vertical blue lines represent periods of non-deposition or missing geological record; open circles: basal conglomerates; vvv: basaltic volcanic rocks. Individual Pb–Zn deposits are shown as yellow stars: 1: Mount Isa; 2: Lady Loretta; 3: McArthur River; 4: Century.
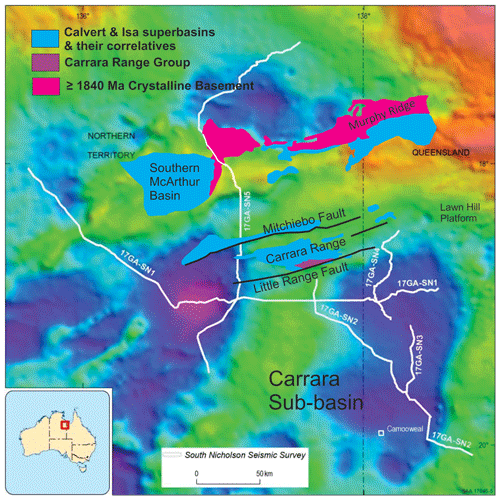
Figure 4South Nicholson seismic grid and late-Paleoproterozoic–early-Mesoproterozoic basinal sequences draped over gravity image for western extension of the Lawn Hill Platform into the Northern Territory. Note anomalously deep gravity low centred on the Carrara Sub-basin (Carr et al., 2019), which is bounded by the Carrara Range (gravity high) to the north of line 17GA–SN1. Geology modified from Rawlings et al. (2008) and Ahmad and Munson (2013). Gravity image reproduced with permission under the Creative Commons Attribution 4.0 International License: http://creativecommons.org/licenses/by/4.0/legalcode. © Commonwealth of Australia (Geoscience Australia) 2020.
The Leichhardt Superbasin concluded in a period of renewed tectonic instability variously attributed to the onset of a 1730–1710 Ma orogenic event (Blaikie et al., 2017) or a renewal in fault-block rotation and tilting (Gibson et al., 2012, 2008). Either way, uplift, and erosion accompanying this event resulted in the formation of a deeply incised and regionally extensive angular unconformity above which conglomerates and redbeds of the Bigie Formation were deposited (Fig. 3). Their deposition marks the start of the 1730–1640 Ma Calvert Superbasin in the Mount Isa region (Figs. 2 and 3) and corresponds to a resumption in backarc extension, bimodal magmatism, and rift-related sedimentation across northern Australia (Gibson et al., 2016; Jackson et al., 2000; Southgate et al., 2000a). Both NW–SE and NE–SW extensional directions have been proposed for the Calvert Superbasin (Fig. 3), and questions remain about the primary orientation of the half-graben hosting the bulk of the basin fill. In the McArthur Basin, this includes rocks of the 1730–1720 Ma basaltic Peters Creek Volcanics and Top Rocky Rhyolite (Page et al., 2000; Rawlings et al., 2008), whereas farther afield on the Lawn Hill Platform (Fig. 1a), the Calvert Superbasin hosts basalts of the 1710–1705 Ma Fiery Creek Volcanics (Fig. 3) and fluviatile to shallow-marine sediments of the 1700–1690 Ma Surprise Creek Formation (Big and Prize supersequences; Southgate et al., 2000a). At about the same time that these rocks were being laid down across the Lawn Hill Platform, water depths began to substantially increase farther east so that by 1690 Ma, basaltic magmas were being extruded and/or intruded into a deep marine basin filled with turbidites (Black et al., 1998; Foster and Austin, 2008; Gibson et al., 2018, 2012; Giles et al., 2002; Glikson et al., 1976; Neumann et al., 2009; Rubenach et al., 2008; Scott et al., 2000; Withnall, 1985). Basaltic magmatism continued through to ca. 1655 Ma in the east, by which time the Leichhardt Superbasin and lower parts of the Calvert Superbasin west of the Leichhardt River fault trough (Fig. 1a) had been intruded by 1680–1670 Ma A-type granites (Sybella Granite) (Neumann et al., 2006) and partially unroofed on top-to-the-northeast extensional shear zones (Gibson et al., 2008).
With the conclusion of bimodal magmatism at 1655 Ma, if not earlier at 1670 Ma in the west, the Calvert Superbasin transitioned from backarc basin to passive rifted continental margin (Baker et al., 2010; Gibson et al., 2018, 2012; Neumann et al., 2009) and began to cool and subside, precipitating a marine transgression during the course of which the North Australian Craton was buried beneath a post-rift sequence (Gun–Loretta supersequences; Fig. 3) of thin-bedded turbidites, carbonaceous shales, black dolomitic siltstones, and carbonate rocks that extended westwards as far as the McArthur Basin (McArthur Group; Figs. 1 and 3) and Lawn Hill Platform (Betts et al., 2016, 2006; Gibson et al., 2012, 2017; Southgate et al., 2013; Withnall and Hutton, 2013). Passive margin conditions persisted until ca. 1650 Ma, by which time northern Australia was subjected to crustal shortening and a further episode of basin inversion (Fig. 3) that lasted until at least 1640 Ma (Riversleigh Tectonic Event) and brought sedimentation in the Calvert Superbasin to a close (Gibson et al., 2018, 2017; Hinman, 1995; Withnall and Hutton, 2013).
Thereafter, the tectonic environment fundamentally changed and crustal extension resumed in a north–south direction (Fig. 3), giving rise to the 1640–1575 Ma Isa Superbasin (Fig. 2) and the deposition of a further 6–8 km of turbiditic sandstones, carbonaceous shales, and dolomitic siltstones (River and Term Supersequences) in fault-bounded basins, predominantly oriented ENE–WSW (Bradshaw et al., 2000, 2018; Gibson et al., 2020; Gorton and Troup, 2018). Despite the resumption in crustal extension, basaltic rocks are absent, and, save for a few tuff beds and rare 1620 Ma rhyolite sills, there was no corresponding resurgence in felsic magmatism until after crustal shortening had largely concluded at ca. 1590–1580 Ma, some 50–60 Ma later (Black and McCulloch, 1990; Gibson et al., 2018; Withnall and Hutton, 2013). The absence of any significant magmatism is in stark contrast to the two older superbasins, leading some researchers to conclude that the Isa Superbasin represents a sag basin, albeit one periodically punctuated by crustal extension (Betts et al., 2003, 2006), whereas others have argued for deposition in a foreland setting (McConachie and Dunster, 1996), pull-apart basin (Scott et al., 1998, 2000; Southgate et al., 2000a), or syn-orogenic basin in which extension was ongoing and facilitated by orogen-parallel strike-slip faulting and lateral extrusion of continental crust (Gibson et al., 2020, 2017). This episode of orogenesis concluded at ca. 1590 Ma (Gibson et al., 2020) or possibly as late as 1580 Ma (Pourteau et al., 2018) before being followed by further crustal extension and successive episodes of pluton-enhanced low-pressure–high-temperature metamorphism at 1560–1540 Ma and 1520–1490 Ma (Duncan et al., 2011; Foster and Rubenach, 2006; Rubenach et al., 2008). Granitic rocks associated with this late metamorphism have both A- and S-type compositions and are mainly to be found in the east, where they are demonstrably of post-tectonic origin, truncating and cutting across folds and axial plane fabrics produced during the Isan Orogeny (Foster and Austin, 2008; Giles et al., 2006; Page and Sun, 1998; Pollard and McNaughton, 1997; Pollard et al., 1998; Withnall and Hutton, 2013).
Granite magmatism concluded in the east at ca. 1500–1490 Ma to be followed by further uplift and erosion across the region before the older sequences were successively buried beneath younger cover rocks of the Mesoproterozoic South Nicholson, Cambrian Georgina, and Mesozoic Carpentaria basins (Sweet, 2017). Remnants of these three younger basins are still widely preserved across the Lawn Hill Platform, extending westwards across the Queensland border into the Northern Territory (Fig. 1a), where the South Nicholson Basin is thickest and occupies several different depocentres, including the Carrara Sub-basin (Fig. 4), for which a large volume of high-quality seismic reflection data has recently become available (eCat: http://pid.geoscience.gov.au/dataset/ga/116881, last access: 2019). Although originally acquired as part of a larger study on the South Nicholson Basin (Carr et al., 2019), these data also serve as a window on the structural architecture of the older underlying basinal sequences, and it is to this that we now turn.
Survey lines for seismic reflection data acquired across the Lawn Hill Platform and already in the public domain are shown in Fig. 5. Most of these are legacy lines dating back to the late 1980s and early 1990s (Burketown Survey, Comalco) (McConachie et al., 1993) and for which reprocessed data became available in 2014 (Armour Energy). Other lines (Fig. 6a and b) were acquired by the minerals industry (Teck Resources, 2011) or minerals industry in collaboration with state and federal governments (Zinifex, Geological Survey of Queensland and Geoscience Australia, 2006), interpretations of which can be found in several publications and government records (Bradshaw et al., 2018; Bradshaw and Scott, 1999; Gibson et al., 2017, 2016; Krassay et al., 2000b; Scott et al., 1998; Southgate et al., 2000b). Results and interpretations of the more recent 2017 South Nicholson Basin survey (Fig. 4) were published jointly by Geoscience Australia and the geological surveys of Queensland and the Northern Territory (Carr et al., 2019), although their interpretation of geology beneath the Carrara Sub-basin (Fig. 4) is not exactly the same as the one presented here, in part owing to uncertainties in extrapolating stratigraphy from existing seismic lines into areas of little or no outcrop or exploratory drilling. The nearest exposures of older rocks are to be found in the Carrara Range immediately north of seismic line 17GA–SN1 (Fig. 4), where a few isolated outcrops of ≥1850 Ma basement schist (Kositcin and Carson, 2019) are overlain by an equally poorly exposed sequence (Carrara Range Group) of sandstones and highly altered basaltic rocks (Mitchiebo Volcanics) long regarded as correlatives of the 1780–1775 Ma Seigal Volcanics in the southern McArthur Basin (Ahmad and Munson, 2013; Rawlings et al., 2008; Sweet, 1984) and Eastern Creek Volcanics in the Leichhardt Superbasin (Fig. 3). The Carrara Range Group has been extensively intruded by the 1725 Ma Top Rocky Rhyolite (Jackson et al., 2000; Page et al., 2000) and is unconformably overlain by sedimentary rocks of equivalent age to the Isa Superbasin (Ahmad and Munson, 2013; Rawlings et al., 2008; Sweet, 1984).
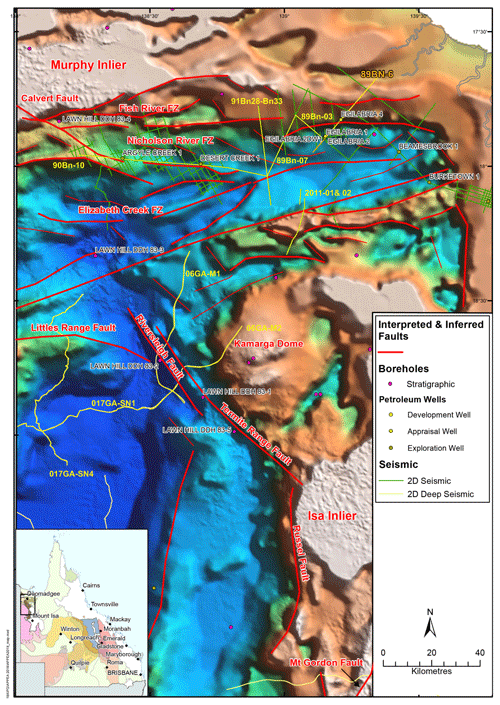
Figure 5Basin and basement architecture for northern Lawn Hill Platform showing the main depocentres, fault trends, and depth to magnetic basement for the Isa Superbasin (after Gorton and Troup, 2018, from image created by Frogtech Geoscience, 2018). Seismic lines are shown in various colours and include the 1994 (Comalco) and 2011 (Teck Resources) industry surveys across the Isa Superbasin and adjacent Punjaub Structure (P). Yellow lines are for composite image presented in Figs. 6–7. Note the compartmentalization of depocentres brought about by interference between the ENE- and NW-trending faults; the latter are of Calvert age and include older normal faults (e.g. Riversleigh Fault) reactivated as strike-slip structures during crustal extension accompanying formation of the Isa Superbasin. The figure is reproduced with permission under the Creative Commons Attribution 4.0 International Licence: http://creativecommons.org/licenses/by/4.0/legalcode. © State Government of Queensland (Geological Survey of Queensland, Georesources Division, Department of Natural Resources, Mines and Energy) 2020.
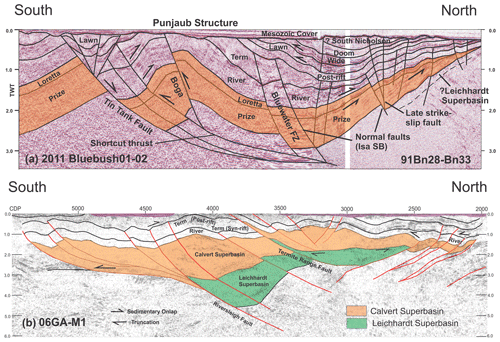
Figure 6North–south-oriented transect across Lawn Hill Platform made up of selected industry and government seismic sections (Fig. 5) showing the predominance of north-dipping normal faults on which there have been successive episodes of basin inversion, leaving behind a legacy of fault reactivation and periclinal folding in (a) Punjaub Structure and (b) Mount Caroline and Ploughed Mountain (after Gibson et al., 2017, 2020). Note the thinning of River and Term supersequences northward through onlap in (a); the underlying Loretta Supersequence similarly thins northward but is bounded top and bottom by truncated surfaces thought to reflect considerable loss of stratigraphic section by uplift and erosion accompanying the 1650–1640 Ma Riversleigh Tectonic Event. Sedimentary patterns point to growth faulting on north-dipping structures during the deposition of the Isa, but not underlying the Calvert Superbasin, whose sequences are merely offset. Bluewater, Boga, and Tin Tank Fault names adopted from Bradshaw et al. (2018) and unpublished industry maps (Pursuit Minerals, 2017). In (b) the older sequence had already been folded before the River Supersequence was deposited as evidenced by the thinning of the latter over the crests of folds developed in the Calvert Superbasin at deeper levels beneath Mount Caroline. The Calvert Superbasin is, in turn, separated by an angular unconformity from an even older underlying sequence inferred (Gibson et al., 2017, 2016) to be the Leichhardt Superbasin. Colour coding is the same as Fig. 3. TWT: two-way time (in seconds; 1 s approximately equals 3 km depth).
In a further departure from previously published interpretations of existing seismic data (Gibson et al., 2017), the Calvert and Isa superbasins are both thought here to encompass discrete syn- and post-inversion sedimentary fractions (Fig. 3). These broadly conform with the sedimentary units or supersequences previously identified at the top of each superbasin (Bradshaw et al., 2000, 2018; Domagala et al., 2000; Krassay et al., 2000a, b; Southgate et al., 2000a) and have an important bearing on basin evolution and more particularly on the timing and duration of the basin inversion events that brought successive basin cycles to a close. These and other differences with previously published interpretations can be illustrated with a few well-chosen survey lines, and there is no need to include interpretations of the full seismic dataset. All lines chosen here are composite and make for two orthogonal but not completely continuous transects across the Lawn Hill Platform, Carrara Range, and neighbouring Carrara Sub-basin (Figs. 4 and 5). For an alternative and slightly different interpretation of these and other lines in the dataset, the reader is referred to Bradshaw and Scott (1999), Bradshaw et al. (2018), and Carr et al. (2019).
3.1 North–south seismic transect across Lawn Hill Platform
This composite transect is made up of several segments (Fig. 6a and b) oriented at high angles to the dominant ENE trend of the Isa Superbasin (Fig. 5). Collectively, these segments image a variably inverted southward-thickening sedimentary wedge disrupted by north-dipping faults and bounded at its top and bottom by major unconformity surfaces. Limited outcrop across the Lawn Hill Platform and ties to cross lines for which oil well stratigraphic data are available (Figs. 5 and 7) would further suggest that the greater part of this wedge comprises rocks of the Calvert and Isa superbasins (Bradshaw et al., 2000; Bradshaw and Scott, 1999; Gorton and Troup, 2018; Scott et al., 1998; Southgate et al., 2000a), although the former is missing its full complement of sedimentary units, having lost the Gun Supersequence and a significant amount of the underlying syn-rift package to erosion so that the Loretta Supersequence now rests directly on a severely truncated Prize Supersequence (Fig. 6a). The Loretta Supersequence is itself truncated beneath the River Supersequence and thins northward through onlap onto what remains of the underlying Prize Supersequence (Fig. 6a). For this part of the Lawn Hill Platform, as much as 1700 m of sedimentary section is estimated to have been removed by erosion from beneath the River Supersequence (Bradshaw et al., 2000), the greater part of which may have been redeposited farther south in the Leichhardt River fault trough (Fig. 1a) where there was a commensurate influx of quartz sand at or before 1640 Ma (Southgate et al., 2000b) during the closing stages of the Calvert Superbasin (Gibson et al., 2017). Basin inversion and an increase in tectonic activity during and subsequent to the deposition of the Loretta Supersequence have been invoked as the most likely cause of this erosion (Bradshaw et al., 2000; Gibson et al., 2020, 2017; Scott et al., 1998), consistent with the observation (Fig. 6a) that this unit is bounded top and bottom by angular unconformities and was laid down at a time of profound change as the depositional environment increasingly began to favour siliciclastic over carbonate sedimentation (Southgate et al., 2000b). Basin inversion at this time is further supported by an increase in redeposited carbonate rocks towards the top of this same supersequence (Southgate et al., 2000b), which, in turn, pass upwards into coarse sandstones and conglomerate (Shady Bore Quartzite), marking not only the base of the unconformably overlying River Supersequence but the start of the Isa Superbasin (Gibson et al., 2017). Similar unconformities have been reported in sequences of comparable age from the southern McArthur Basin (Kunzmann et al., 2019; Rawlings et al., 2008), indicating that this phase of basin inversion, uplift, and erosion is more widely developed across northern Australia and is not confined to the Lawn Hill Platform. Conversely, even though rocks of equivalent age to the Leichhardt Superbasin occur widely across the McArthur Basin (Tawallah Group; Fig. 3) as well as farther south in the Leichhardt River fault trough (Fig. 1a), they are either missing from the seismic sections investigated here (Fig. 6a and b) or reduced to a thin layer sandwiched between basement and the overlying younger basins (cf. Scott et al., 1998). Seismic reflections below the level of the Prize Supersequence are too poorly resolved for us to be confident that the rocks in question belong to one or the other of the two superbasins.
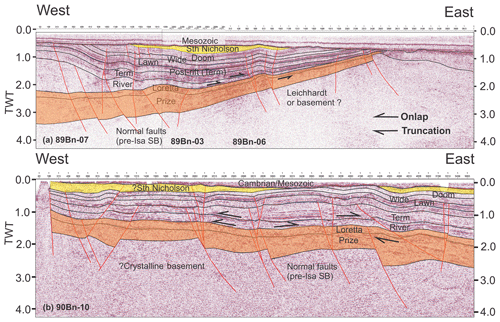
Figure 7West–east-oriented seismic transects through northern Lawn Hill Platform. Westward thickening of Calvert-age sedimentary units in both (a) and (b) is consistent with growth faulting on east-dipping normal faults which have since been variably reactivated (Isa Orogeny). Conversely, no such growth is obviously developed in the overlying Isa Superbasin, whose lithological strike and inversion structures are essentially parallel to the line of section. These units still thicken into the centre of the Isa Superbasin but in a direction orthogonal to normal faults active during its deposition. Such faults, if imaged, would be expected to be flat-lying or have very shallow dips parallel or subparallel to bedding in both sections, rendering their recognition very difficult. Some of the disruption to bedding in (b) along line 90Bn10 may be due to such faults, but overall the most obvious structural feature in the section is the broad arching and folding of stratigraphy in the middle of the section consistent with imaging of an inversion fold in longitudinal cross section. Note truncated surface and angular unconformity at the base of Loretta Supersequence in both sections which is interpreted here to be an expression of basin inversion linked to the 1650–1640 Ma Riversleigh Tectonic Event.
In marked contrast, all five supersequences of the Isa Superbasin (Fig. 3) are well imaged along Comalco line 91Bn33–91Bn28, attaining a maximum thickness of 5–6 km (Fig. 6a). Basin architecture along this particular line was first described in detail by Bradshaw et al. (2000), and their interpretation is not dissimilar to the one presented here. More specifically, as in Bradshaw et al. (2000), several inverted normal faults are recognized, into which both the River and Term supersequences manifestly thicken (Fig. 6a). Normal faults of this age dip northward and even though some disrupt and offset sedimentary units in the underlying Calvert Superbasin (Loretta and Prize supersequences), there is no reason to conclude that any of these structures ever served as growth faults during the deposition of the older sedimentary basin. Rather, these north-dipping faults cut across and postdate stratigraphy in the Calvert Superbasin and first became active during the deposition of the Isa Superbasin. They include the ENE-striking Bluewater and Tin Tank faults (Bradshaw et al., 2018; Pursuit Minerals, 2017), both of which dip to the north and were reactivated in the opposite sense during basin inversion (Fig. 6a). The more steeply dipping Boga Fault (Pursuit Minerals, 2017) may similarly have been reactivated at this time but does not share the same dip or strike as the other two structures. It intersects the seismic section at nearly 90∘ and has a northwest strike more in keeping with other faults of Calvert age across the region (e.g. Riversleigh Fault; Fig. 5). Moreover, unlike the Bluewater and Tin Tank faults, this structure is associated with a footwall shortcut thrust that not only accommodated a significant amount of strain during basin inversion but shares the same geometry as a similar structure developed in the footwall of the equally steeply dipping Riversleigh Fault farther south (Fig. 6a). Importantly, neither this footwall thrust nor any of the reactivated normal faults have effected displacements large enough so as to completely disrupt stratigraphy. Instead, stratigraphy continues southward into the hangingwall of the Tin Tank Fault (Fig. 6a) where the Calvert and Isa superbasins have been spectacularly deformed into a kilometre-scale, south-verging antiformal fold (Punjaub Structure). The River and Term supersequences attain maximum stratigraphic thickness in the core of this fold, which is both strikingly asymmetric in character and by far the most obvious inversion structure developed along this particular segment of the north–south transect (Fig. 6a).
Conversely, through a combination of onlap and truncation, the Lawn and Wide supersequences both lose thickness over the crest of this same antiformal fold (Fig. 6a). Despite the loss of sedimentary record and even more obvious truncation at the base of the overlying Mesozoic cover rocks (Fig. 6a), this thinning appears to be an original depositional feature with neither sedimentary unit ever having maintained constant stratigraphic thickness across the fold. Instead, their sedimentary thickness was strongly influenced by the presence of this structure. It follows that the Punjaub Structure either already existed by the time the Lawn and Wide supersequences were deposited or the fold was actively growing during the course of their deposition. Irrespective of which interpretation is correct, these two units cannot be part of the syn-extensional growth package. Rather, extension in the Isa Superbasin had already come to a close before sedimentation ceased, and both units more rightly belong to the post-rift or syn-inversion fraction of basin fill. A similar conclusion was reached by Gibson et al. (2017) for the Lawn and Wide supersequences exposed farther south along seismic line 06GAM2 in the Century region (see below). Basin architecture in these two regions shares many similarities, not least of which is a folded and locally inverted basin fill which, in the Century region, is known to host a world class Pb–Zn mineral deposit (Southgate et al., 2000a, 2006). Onlap of the River Supersequence onto older folded units in the cores of the doubly plunging Mount Caroline and Ploughed Mountain folds (Figs. 1b and 6b) would further suggest that this was not the only episode of basin inversion to have affected the rocks of the Lawn Hill Platform. However, as elsewhere across northern Australia, the existence of the older deformational event has largely gone unrecognized owing to extensive overprinting during the 1620–1580 Ma Isa Orogeny and at least one additional shortening event that postdates the Isa Orogeny and folded all units up to and including the South Nicholson Basin (Fig. 6a). Doubly plunging periclinal folds like Ploughed Mountain and Mount Caroline (Figs. 1b and 6b) may similarly be an expression of this younger event, although, as already mentioned, they more likely first came into existence during the earlier basin inversion event and were subsequently reactivated and/or tightened during later deformation.
3.2 West–east seismic transect through the Calvert and Isa superbasins
As with the north–south transect, seismic sections oriented west–east or northeast–southwest are dominated by rocks of the Calvert and Isa superbasins and share the same wedge-like geometry (Fig. 7a and b). This wedge-like geometry is particularly well illustrated in composite section 89Bn07–89Bn03–89Bn06 and shows the Isa Superbasin thickening from east to west with the main sedimentary depocentre located towards the southwest as might be expected in a seismic section oriented parallel or subparallel to basin strike. Absent or difficult to recognize in either this section or 90Bn10 (Fig. 7b) are structures that might have unequivocally served as growth faults during the development of the Isa Superbasin. Normal faults of this age dip predominantly northward (Fig. 6a) and as such are anticipated to have shallow-dipping or subhorizontal traces in west–east-oriented seismic sections but still cut up-section all the way to the Term Supersequence. Few structures with the requisite shallow dips have been identified in either section, and the only evidence of structural control on basin fill occurs at deeper levels in the Calvert Superbasin where the Loretta and Prize supersequences both thicken into northeast-dipping structures (Fig. 7b). Although locally disrupted by later subvertical faults, these structures demonstrably served as growth faults during the deposition of the Calvert Superbasin and typically cut up-section no farther than the Loretta Supersequence (Fig. 7b). However, like the Riversleigh Fault (Fig. 8a) and other faults sharing the same northwest trend on the Lawn Hill Platform, these structures were subsequently reactivated in an oblique sense and continued to act as growth faults during the deposition of the younger River and lowermost Term supersequences (Fig. 8a).
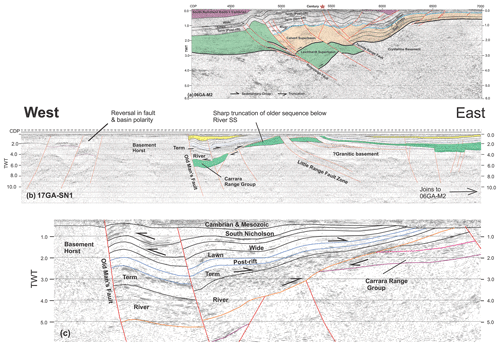
Figure 8West–east-oriented section through (a) Century Mine (06GAM2); (b) its western continuation (17GA–SN1) across the South Nicholson Basin and Carrara Sub-basin (Carr et al., 2019); and (c) enlarged section of 17GA–SN1 showing angular unconformity between the Isa Superbasin and an underlying, variably truncated older basin sequence. This older basin sequence directly overlies crystalline basement and likely comprises rocks of the Leichhardt Superbasin, correlatives of which (Carrara Range Group) are exposed in the Carrara Range immediately to the north of seismic line 17GA–SN1 (see Fig. 4). Note also that this older sequence is contiguous with rocks in the Century region (06GA-M2) previously identified as part of the Leichhardt Superbasin (Gibson et al., 2017). Conversely, the Calvert Superbasin is missing west of the Riversleigh Fault consistent with non-deposition or a period of uplift and erosion during the course of which it and a significant amount of the underlying Leichhardt Superbasin were removed from the geological record. Note that only a portion of line 17GA-SN1 is presented and interpreted here (cf. Carr et al., 2019, who present an interpretation of the full line)
Following the deposition of the River and lowermost Term supersequences, extension and normal faulting across the Lawn Hill Platform ceased and the syn-rift fraction of basin fill was buried beneath a post-rift blanket of near-constant thickness that comprises the rest of the Term Supersequence (Fig. 7a and b). This was superseded, in turn, by the deposition of the Lawn, Wide, and Doom supersequences, which, together, make up the syn-inversion package and conspicuously thin over the crest of folds developed in the hangingwalls of inverted normal faults such as the Tin Tank and Riversleigh structures (Figs. 6a and 8a). The thinning of the younger stratigraphic units across the crests of antiformal folds is no less conspicuous in the west–east-oriented seismic sections shown here and is particularly evident where the underlying syn-rift sequence attains maximum thickness and has undergone the greatest amount of inversion-related uplift (Fig. 7a and b). Such relationships are not unexpected and conform to theoretical expectations for inverted sedimentary basins (Cooper et al., 1989). They also lend support to the idea that basin inversion, and deformation more generally, had already commenced across the Lawn Hill Platform before sedimentation in the Isa Superbasin had come to a close (Gibson et al., 2017).
Conversely, the few large faults cutting upwards through these younger stratigraphic units from deeper levels in the Isa or Calvert superbasins are too steeply dipping to be reactivated normal faults or inversion-related structures and more likely represent strike-slip faults. Their age is not well constrained, although some cut up-section as far as the South Nicholson Basin and evidently formed late. Others terminate at deeper stratigraphic levels and may be more like the Riversleigh Fault (Fig. 8a), which was reactivated in a strike-slip sense during and subsequent to the onset of north–south extension and concomitant deposition of the River and Term supersequences (Gibson et al., 2017).
3.3 Basin inversion west of the Lawn Hill Platform
Seismic reflection data collected west of the Lawn Hill Platform with a view to better understanding the mineral and hydrocarbon potential of the poorly exposed Mesoproterozoic South Nicholson Basin, and the Carrara Sub-basin in particular (Fig. 4), have already been interpreted and published in their entirety (Carr et al., 2019), and this paper is only concerned with parts of the dataset that may be used to shed further light on basin architecture in the underlying older sequences. Especially pertinent in this regard are the constraints imposed by the eastern half of seismic line 17GA–SN1 (Fig. 8b) which joins onto the existing Century line (06GAM2) just west of the Riversleigh Fault (Figs. 5 and 8a). As such, these two lines make for a single continuous and greatly expanded west–east transect through this part of northern Australia and might be expected to share a common older geology and basin architecture (Fig. 8b). An interpretation of these two lines is presented in Fig. 8a–c and would appear to confirm that this is indeed the case for the younger post-rift and syn-inversion fractions of basin fill in the Isa Superbasin. These two fractions not only continue without break from one seismic line into the other but can be traced westwards beneath the Carrara Sub-basin for tens of kilometres before eventually thinning over a basement high located just south of the Carrara Range (Fig. 4) where seismic lines 17GA–SN1 and SN2 intersect (see also Carr et al., 2019). This basement high developed in the hangingwall of a major south- or southeast-dipping fault or fault zone (Little Range Fault Zone) but owing to the west–east orientation of the seismic profile (Fig. 4), this structure and its hangingwall sequences are imaged in oblique section and thus dip far less steeply than in other sections oriented at higher angles to fault strike (e.g. 17GA–SN2 and 17GA–SN4). Basement rocks, along with the rest of the hangingwall sequence, have nevertheless been clearly inverted and take the form of a broad arch or subdued fold in the seismic image (Fig. 8b). No less importantly, from the thinning of younger sedimentary units onto the eastern flank of this fold (Fig. 8b), it may be further deduced that basin inversion, as in the Punjaub Structure, was already underway before the last of the Isa Superbasin had been deposited.
Thinning of the younger stratigraphic units aside, it is equally evident from the seismic data that the hangingwall of the Little Range Fault Zone comprises a less than complete sedimentary record (Fig. 8b). The syn-rift fraction of basin fill (River and lowermost Term supersequences) and any unit that might be reliably identified as part of the Calvert Superbasin are missing and do not appear to extend any farther west than the Riversleigh Fault (Fig. 8a and b). Particularly conspicuous by its absence is the Loretta Supersequence, whose transparent to weakly reflective character in seismic sections (Bradshaw et al., 2000; Southgate et al., 2000a) is usually enough to distinguish it from other stratigraphic units in the Calvert and Isa superbasins (e.g. Figs. 6–7). Either this and the other missing sedimentary units were never deposited west of the Riversleigh Fault or, as elsewhere across the region, these rocks have been removed by erosion so that younger elements of the Isa Superbasin have come to directly overlie older rocks thought (Gibson et al., 2017) to form part of the Leichhardt Superbasin (Fig. 8a and b). Stratigraphy in this older basin is variably truncated beneath rocks of the overlying Isa Superbasin and, like them, can be traced westwards for some distance towards the Little Range Fault Zone and its basement high, over the top of which the older rocks almost reach the surface (Fig. 8b). Nowhere, however, along the seismic line are the older rocks actually exposed. A thin veneer of Cambrian and/or Mesozoic sediments (Georgina and Carpentaria basins) always intervenes, although rocks long thought (Ahmad and Munson, 2013; Rawlings et al., 2008) to be correlatives of the Leichhardt Superbasin (Fig. 3) are exposed north of the seismic line in the Carrara Range (Carrara Range Group: Fig. 4). Moreover, these rocks have since been shown to have the same age as the Leichhardt Superbasin based on strikingly similar detrital zircon populations (Kositcin and Carson, 2019). They also rest unconformably on ≥1840 Ma psammopelitic schists which not only represent basement to the Carrara Range Group in this region (Ahmad and Munson, 2013; Rawlings et al., 2008) but likely serve as a good proxy for unexposed basement along the seismic line a short distance to the south.
West of the Little Range Fault Zone is a second, even larger uplifted basement block or horst bounded on its eastern side by a steeply dipping fault and inverted sedimentary basin (Fig. 8c) filled almost entirely by rocks of the Isa Superbasin. Basin fill is up to 10 km thick and strikingly similar in both volume and geometry to the correlative sequence exposed in the hangingwall of the Riversleigh Fault farther east (Fig. 8a). As with the latter, this includes the full complement of post-rift and syn-inversion sedimentary units (Lawn, Wide, and Doom supersequences) as well as a thicker syn-rift package of more strongly reflective rocks interpreted here (Fig. 8c) to be made up of the River and Term supersequences. The River Supersequence is particularly well represented, thickening into the Old Man's Fault (informal name) and characteristically exhibiting a wedge-like geometry that thins eastwards through overlap onto the adjacent basement high and its cap of the Leichhardt Superbasin (Fig. 8c). Its contact with the underlying older rocks just west of the Little Range Fault Zone is an angular unconformity beneath which the Leichhardt Superbasin is abruptly truncated or lost altogether so that the Isa Superbasin rests directly on the underlying basement (Fig. 8c). Significantly, this same angular relationship has been observed in outcrop in the Carrara Range (Sweet, 1984) where rocks of the McNamara Group (Isa Superbasin) directly overlie the Carrara Range Group (Leichhardt Superbasin equivalents). It is thus not unreasonable to conclude that the River Supersequence was deposited on an already uplifted and eroded surface that cuts downward ever more deeply in a southerly or east-to-west direction so as to expose progressively older elements of the regional stratigraphy in and around the Carrara Range. Moreover, even though the bulk of this uplift and erosion likely occurred during the 1650–1640 Riversleigh Tectonic Event immediately prior to the deposition of the River Supersequence, the possibility that some of it dates from an even older 1730 Ma episode of deformation better known from the McArthur Basin as the mid-Tawallah Compressional Event (Ahmad and Munson, 2013) cannot be excluded. Notwithstanding such uncertainties, basement and its bounding faults clearly played an important role in controlling basin architecture and continued to be active long after the deposition of the Isa Superbasin had concluded as both the South Nicholson and Georgina basins similarly thin across them (Fig. 8c). Equally notable in this context is an obvious reversal in basin and fault polarity on either side of the larger basement horst block (Fig. 8b).
3.4 North–south seismic sections orthogonal to 17GA–SN1
As a further check on basin geometry and structural architecture to the west of the Lawn Hill Platform, two sections (17GA–SN2 and 17GA–SN4) were selected for interpretation in a direction at a high angle or orthogonal to 17GA–SN1 (Fig. 9a and b). With its NW–SE orientation, seismic line 17GA–SN2 cuts across many of the same structures imaged in 17GA–SN1, most notably at least one crustal-scale south- or southeast-dipping shear zone or fault system that extends downward into the lower crust and possibly as far as the MOHO (Figs. 8b and 9a). This shear zone is several hundred metres wide and is likely the same reactivated basement structure identified as the Little Range Fault Zone along line 17GA–SN1; it has variably deformed rocks of the Leichhardt and Isa superbasins in its hangingwall (Fig. 9a) and appears to have served as a thrust system during basin inversion, effecting upward displacement not only of the former but the underlying basement rocks. The most obvious difference between lines 17GA–SN1 and 17GA–SN2 is the thick wedge-like basinal sequence imaged down to 7.0 s TWT in the hangingwall of this same shear zone (Fig. 9a). This basinal sequence lies below the interpreted base of the Leichhardt Superbasin in line 17GA–SN1 (Fig. 8b) and either represents an entirely separate older volcanic or sedimentary package or a continuation of the Leichhardt Superbasin downward to much greater depths than is immediately apparent in the west–east-oriented seismic section. In the absence of independent information, it is not possible to discriminate between these two possibilities except to say that the same package is evidently present in line 17GA–SN1 and corresponds to a zone of non-reflective crust which at a depth of 10 km or more seems much too deep for the Leichhardt Superbasin (Fig. 9a) and shares none of the higher-amplitude reflectors common to other seismic lines through this basin elsewhere in the Mount Isa region (e.g. 06GA–M3; Gibson et al., 2016). Several other low-angle faults imaged in lines 17GA–SN1 and 17GA–SN2 also root downward into this zone (Figs. 8b and 9a), although neither they nor the surfaces bounding the older sedimentary package are particularly conspicuous or maintain their individual character at depth. Rather, all of these faults and surfaces merge downward into one seismically bland and homogenized region of middle crust more in keeping with expectations for metamorphic basement than the Leichhardt Superbasin. Moreover, because line 17GA–SN1 is probably more closely aligned with lithological strike in the older sedimentary package and its bounding surfaces, their apparent dip in this particular seismic section is close to zero, resulting in surfaces whose traces are subhorizontal and parallel to each other. Only along line 17GA–SN2 is the northern dip of this older seismic package discernible.
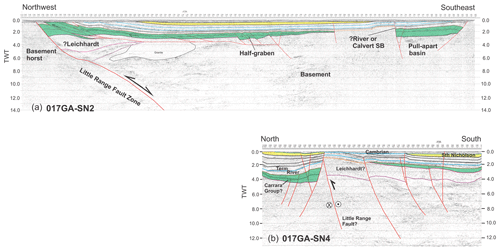
Figure 9North–south-oriented seismic sections west of Lawn Hill Platform showing shallow crystalline basement and the same basement-rooted inversion structure. Note the major basement structure in (a) that cuts downward all the way to the MOHO is probably the same basement structure or fault zone (Little Range Fault Zone) imaged in (b), over which all older sedimentary basins have been eroded so that rocks of Cambrian age (Georgina Basin) directly overlie basement (17GA–SN4). Rocks of the South Nicholson have similarly been removed for the crest of this basement block in 17GA–SN4 and, together with rocks of the Isa Superbasin, increase in thickness northwards. The Isa Superbasin is abruptly truncated by the basement structures and other subvertical structures suggestive of a flower structure and late-stage onset of strike-slip faulting at or before the deposition of the Cambrian Georgina Basin.
As with the other two seismic lines across the South Nicholson Basin, 17GA–SN4 has imaged a basement block overlain by a southward-thickening wedge of sedimentary rocks mainly belonging to the Isa Superbasin but beneath which there is a variably truncated and faulted older sequence. This older sequence rests directly on basement and likely incorporates correlatives of the Leichhardt Superbasin (Fig. 9b). Seemingly missing again is the Calvert Superbasin and its place has been taken up by a package of rocks (Fig. 9b) whose seismic character (short, high-amplitude reflectors) is a better fit for the River and/or Term Supersequence as opposed to the Loretta or Prize supersequences. Lying above this package are several sedimentary units identified elsewhere in this paper as belonging to the post-rift and syn-inversion fractions of basin fill in the Isa Superbasin. They, in turn, are overlain by younger sediments of the South Nicholson and Georgina basins, although the latter is very thin and represents no more than a veneer over the underlying rocks (Fig. 9b).
Importantly, essentially the same stratigraphic package can be identified north of the seismically imaged basement block, except that all sedimentary units, including those making up the South Nicholson Basin, are appreciably thicker and have a different geometry, pointing to deposition in a separate sub-basin to the Carrara Sub-basin (Fig. 9b). In keeping with this interpretation, the two depocentres are separated by a major fault which dips steeply south and is likely an along-strike continuation of the same south-dipping fault system (Little Range Fault Zone) imaged farther west along seismic lines 17GA–SN1 and SN2. As with the other two structures, this fault played an equally important role in basin formation and was reactivated on more than one occasion because sedimentary units in its hangingwall, up to and including the South Nicholson Basin, have all been inverted, whereas older rocks in its footwall are cut by this basement fault and terminate abruptly against it. This includes rocks of the River and/or Term supersequences which were deposited on an older and already folded older sequence taken here to be the Paleoproterozoic Carrara Range Group (Fig. 9b) and thus part of the Leichhardt Superbasin (Fig. 3). No less conspicuously, this older sequence thickens towards basement and its bounding fault, whereas sedimentary sequences making up the overlying South Nicholson Basin and higher levels of the Isa Superbasin (Lawn through to Doom supersequences) do the opposite; they instead thin towards this basement high and likely onlapped the latter at the time these rocks were being deposited. The seismic images are consistent with such an interpretation, particularly for the higher-level sedimentary units (Fig. 9b), which still show significant amounts of thinning over the adjacent basement high despite successive episodes of fault reactivation. Sedimentation, paired with uplift and erosion, has been observed before in the Paleo–Mesoproterozoic basins of northern Australia and is usually attributed to repeated episodes of rift-sag (Betts et al., 2016, 2003, 2006; O'Dea et al., 1997b) or strike-slip faulting along basin-bounding structures (Scott et al., 1998; Southgate et al., 2000b). Basin inversion with a few notable exceptions (Blaikie et al., 2017; Broadbent et al., 1998; Gibson et al., 2017) was largely treated as a late or secondary process and thus incidental to the formation of Pb–Zn mineral deposits. Interpreted seismic data presented in this paper would suggest otherwise and indicate that basin inversion as a process had a far greater impact on basin history and mineralization than has hitherto been recognized.
4.1 Basin inversion structures: timing and distribution
Inverted extensional basins and their structural architecture have been widely investigated and described following numerous field studies combined with the results of numerical modelling and sandbox experiments (Cooper et al., 1989; Hayward and Graham, 1989; McClay et al., 2002; McClay and White, 1995; Turner and Williams, 2004). Emphasized in most of these studies is the strongly asymmetric nature of the pre-existing basin fill and the consequences of shortening a sedimentary sequence whose individual unit lengths are all different and increase upward from the bottom to the top of the section (Hayward and Graham, 1989; Lowell, 1995; Turner and Williams, 2004). The net result during shortening is the development of an equally asymmetric fold in the hangingwall of the original normal fault, which may or may not have been reactivated during the process. This hangingwall fold is one of the most distinctive features of basin inversion and may be regarded as a diagnostic feature, particularly in cases where folding is enhanced by the reactivation of coeval antithetic structures leading to the expulsion of basin fill in opposite directions. Further enhancements of the basic inverted structure may occur where the normal fault locks up early and strain is transferred to a footwall shortcut thrust or taken up by some other structure such as a strike-slip fault (Dooley and McClay, 1997; McClay, 1995; McClay et al., 2002). These and other variations on structural architecture developed during basin inversion (Martínez et al., 2012) are illustrated in Fig. 10. All examples are from inverted basins of Mesozoic or younger age but are clearly no less relevant in the case of the older basins described here from northern Australia. Footwall shortcut thrusts have been captured in several of the seismic sections but are conspicuously well developed in the footwalls of the Riversleigh (06GA–M2) and Boga structures (Figs. 8a and 6b). However, by far the most common and widely imaged structure is the hangingwall antiform (Fig. 10). Moreover, this same structural feature is evident in all sections irrespective of whether they are oriented north–south or west–east, supporting suggestions made elsewhere that there has been more than one episode of basin inversion and that these were imposed on basins that were originally orthogonal to one another. As such, basin inversion affords clues to basin orientation before and after successive episodes of crustal shortening got underway, and it is to this topic that we now turn.
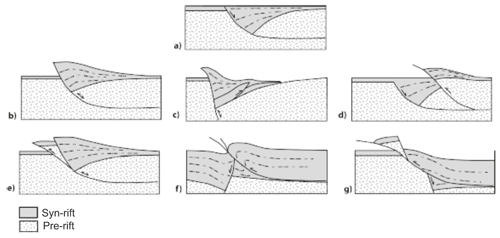
Figure 10Basin inversion and resulting styles of structural architecture to be anticipated during crustal shortening (after Martínez et al., 2012; McClay, 1995): (a) early normal fault; (b) harpoon structure from partially inverted normal fault; (c) buttressing; (d) hangingwall is faulted forming hangingwall shortcut; (e) footwall shortcut thrust; (f) folding and truncation of normal fault by younger thrust; (g) thrust ramp above normal fault.
The Isa Superbasin is best known from the Lawn Hill Platform (Fig. 5) and has been previously interpreted as a sag or foreland basin deformed during a subsequent north–south shortening event identified as the Isan Orogeny (Betts et al., 2003; McConachie et al., 1993; McConachie and Dunster, 1996). More recently, an extensional origin has been proposed for this same basin consistent with seismic data and general thickening of sedimentary units like the River and Term supersequences into normal faults oriented ENE–WSW (Bradshaw et al., 2018; Gorton and Troup, 2018). Antiformal closures developed in the Punjaub Structure and periclinal folds exposed just to its south at Mount Caroline and Ploughed Mountain share the same ENE–WSW trend (Fig. 1b) and likely represent basin inversion structures formed during the same north–south shortening event. However, as already pointed out, the Punjaub Structure is not a simple structure and likely underwent limited folding before or subsequent to the start of deposition in the River and Term supersequences (Gibson et al., 2020). Along with rocks of Calvert age in the core of the Punjaub Structure, these two sequences were deformed during the 1650–1640 Ma Riversleigh Tectonic Event for which a NE–SW shortening direction has been proposed (Gibson et al., 2020, 2017). As such, shortening during the earlier stages of basin inversion in the Punjaub Structure would have been approximately orthogonal to strike in the Calvert Superbasin and its NW–SE basin-bounding normal faults. Seismic sections oriented parallel to this shortening direction consistently show faults of Calvert age dipping eastwards (e.g. Riversleigh Fault), and several have antiformal structures developed in their hangingwalls (Fig. 8a and b) in accord with expectations that basin geometry prior to inversion was highly asymmetric and had the form of a westward-deepening half-graben. Westward deepening of Calvert-age extensional basins on the Lawn Hill Platform is contrary to the results of earlier geophysical modelling (Betts et al., 2004) indicating that half-graben of this age deepen southwards towards normal faults with NE orientations essentially orthogonal to what is proposed here. However, while faults with this orientation have been previously mapped (Hutton and Sweet, 1982) or have been known to exist in the subsurface for a long time (Krassay et al., 2000a; Scott et al., 1998), it is debatable that they are of Calvert age or exercised any significant control on depositional patterns during this phase of basin formation. They share the same NE-to-ENE strike as normal faults in the Isa Superbasin and likely belong to the same generation of structures that controlled the deposition of the younger sedimentary basin. Importantly, faults of this age exhibit increased amounts of throw southwards, which would have been accompanied by commensurate amounts of downward displacement in rocks of the underlying Calvert Superbasin, as captured in seismic images (Fig. 6a) along line 91Bn28–91Bn33 and the northern flank of the Punjaub Structure where the Loretta and Prize supersequences, along with older elements of the Isa Superbasin, have been faulted downward by several kilometres relative to their counterparts across the crest of the fold. This is the same stepped basin geometry picked up in the results of geophysical modelling for the Lawn Hill Platform (Betts et al., 2004) and which, during later crustal shortening, would have produced south-verging folds with the same NE axial direction orientation as periclinal folds now observed at Mount Caroline and Ploughed Mountain (Fig. 1b). It further follows that the NW–SE extensional direction previously proposed for the Calvert Superbasin more likely relates to the younger Isa Superbasin and only came about because erosion across the Lawn Hill Platform during or subsequent to the Isa Orogeny removed much of the younger basin infrastructure leaving behind only the inverted and once more deeply buried rocks of the older basin.
West of the Lawn Hill Platform, crystalline basement lies at much shallower crustal depths and may even have been exposed during deposition of the Georgina or South Nicholson basins, forming one or more structural highs over the top of which there is a conspicuous thinning or draping of the younger cover rocks (Fig. 9b). The older-Paleoproterozoic–early-Mesoproterozoic sequences are similarly notably thinner over basement in this region and the Calvert Superbasin may be entirely missing (Figs. 8b and 9b), either because it was never deposited or was removed by erosion during uplift accompanying the Riversleigh Tectonic Event. The River Supersequence consequently directly overlies a truncated and westward-thinning older sequence taken here to be the Leichhardt Superbasin based on continuity with line 06GAM2 (Fig. 8a), for which a stratigraphic interpretation has already been published. As with line 06GAM2 (Fig. 8a), an angular unconformity separates the two sequences (see also Sweet, 1984), and the Leichhardt Superbasin had likely already been inverted before the River Supersequence was laid down. In keeping with this suggestion, the older sequence has locally been completely eroded away so that the River Supersequence rests directly on older crystalline basement (Fig. 8b). Moreover, even though a significant amount of this uplift and erosion may have been accommodated on reactivated older normal faults, the dominant structures in the seismic images are subvertical to steeply dipping and abruptly truncate stratigraphy not only in basement but thick basinal sequences developed in their footwalls. These footwall sequences encompass most if not all units of the Isa Superbasin (Figs. 8b and 9b), pointing to either a considerable amount of downward throw to the north on these structures or an equally significant amount of strike-slip displacement. The latter is thought more likely here, consistent with the scale and abruptness of truncation and the observation that the faults overall have the character of flower-like structures (Fig. 9b). Such uncertainties aside, it seems reasonable to conclude that basement uplift on these subvertical faults occurred late as these structures displace all units in the Isa Superbasin and cut up-section all the way to the base of the Cambrian. Faults with similarly steep attitudes and character are also evident in north–south-oriented seismic sections (Fig. 6) for the northern Lawn Hill Platform (e.g. 91Bn28–91Bn33) and likely belong to the same generation of strike-slip faults. Significantly, they share the same NE-to-ENE strike and were possibly initiated on older structures dating back to formation of the Isa Superbasin into which they root downward (Fig. 6a). Late NE-trending strike-slip faulting has recently been attributed to the onset of extensional collapse and orogen-parallel extrusion of thermally weakened crust following arc–continent or continent–continent collision between Australia and Laurentia (Gibson et al., 2020).
4.2 Basin inversion and implications for Pb–Zn mineralization
As revealed in seismic sections oriented orthogonal to one another, basin inversion in the north Australian Paleoproterozoic–Mesoproterozoic sequences occurred on more than one occasion and gave rise to a structural architecture not unlike that recorded in basins of much younger age such as the Irish and North seas and North Atlantic petroleum province more generally. No less important in this context are the results of past and recent drilling confirming that northern Australia is prospective for oil and gas (Gorton and Troup, 2018; Jackson et al., 1986; McConachie et al., 1993), not all of which has its source in the South Nicholson or younger sedimentary basins (Glikson et al., 2006; Golding et al., 2006). Some is known to be considerably older (Jackson et al., 1986) or at least date back to the time of Pb–Zn mineralization in the 1575 Ma Century deposit (Broadbent et al., 1998). Bituminous residues occur widely throughout this deposit (Broadbent et al., 1998), and more recent studies have shown that carbonaceous shales and dolomitic siltstones hosting the ore body (Wide Supersequence) contain exceptionally high levels of total organic carbon, along with other parts of the Isa Superbasin (Glikson et al., 2006; Gorton and Troup, 2018; Jarrett et al., 2018). However, this need not imply that the mineralizing fluids were emplaced into an existing oil or gas reservoir as required by the Broadbent et al. (1998) model for ore formation. An alternative possibility is that the organic matter was already present in the host rocks from the time they were deposited and was transformed into oil and/or gas during ingress of the mineralizing fluid itself (Glikson et al., 2006; Golding et al., 2006). Either way, it is difficult to avoid the conclusion that a petroleum system was in operation during or immediately prior to mineralization. Moreover, if fluid:rock ratios were high at the time of mineralization, much of the organic carbon would have been removed from the deposit site, leaving behind bituminous residues but more importantly leading to transient increases in pore space that was subsequently filled by sulfides (Glikson et al., 2006). Stable isotope studies combined with analyses of illite crystallinity and organic reflectance would further indicate that this process occurred under a normal thermal gradient (ca. 24 ∘C km−1) and temperatures below 200 ∘C (Glikson et al., 2006; Golding et al., 2006) and thus without the need for additional magmatic or metamorphic heating. Bimodal magmatism ceased some 60–70 Myr earlier at ca. 1655 Ma, and so any related thermal anomaly would have long since decayed and been unavailable to drive either fluid flow or the mineralization process. Instead, any extraneous heat added to the system may have come from the hydrothermal fluids themselves whose expulsion from deeper levels of the basin was more likely driven by a build-up of fluid overpressures as the twin processes of crustal shortening and basin inversion took effect.
Significantly, a near-identical scenario has been proposed for some carbonate-hosted Pb–Zn deposits of the Mississippi Valley-type (Leach et al., 2001, 2010) which nearly always contain some amount of pyrobitumen or organic carbon and may similarly have formed through mixing of hydrocarbons with a hydrothermal metal-bearing fluid (Anderson, 2008; Kesler et al., 1994). Such similarities with Century and the clastic-dominated Pb–Zn deposits of northern Australia have been noted before (Huston et al., 2006) but are usually tempered by perceived differences in tectonic setting or the timing of mineralization relative to orogenesis. A compressional foreland setting is usually advanced for the former (Leach et al., 2001), whereas the Pb–Zn deposits of northern Australia are thought to be largely syn-extensional in origin and to have formed prior to the onset of orogenesis (Huston et al., 2006; Large et al., 2005). In the interpretation presented here, there is no discernible difference in tectonic setting between the two different deposit styles, and late-Paleoproterozoic–early Mesoproterozoic Pb–Zn mineralization at Century and elsewhere across the region was driven by basin inversion linked to orogenesis and crustal shortening (Gibson et al., 2020, 2017) as originally envisaged by Broadbent et al. (1998).
Moreover, in view of seismic evidence for basin inversion towards the end of Calvert time (e.g. Fig. 6a), there is no reason why the Walford Creek Pb–Zn deposit (Rohrlach et al., 1998) located just to the north of Century (Fig. 1a) could not have formed under similar circumstances. Although older, and hosted by carbonate rocks of the Loretta Supersequence (Walford Dolomite), its host rocks were similarly laid down during a period of increasing tectonic instability (Riversleigh Tectonic Event). The deposit itself has also been described as being of Mississippi Valley-type (Rohrlach et al., 1998). No less importantly, fluid inclusions from this ore body are known to contain light oil (Rohrlach et al., 1998), most likely sourced and introduced ahead of mineralization from shales rich in organic carbon (Mount Les Siltstone) in the overlying River Supersequence (Glikson et al., 2006). Thus, not only were hydrocarbons leaking from one stratigraphic unit into another in this region but there is a strong possibility that a petroleum system was present up to and possibly including the time of mineralization. Accordingly, as at Century, the mineralizing fluid may have interacted or mixed with organic carbon, making for a highly reducing environment into which more oxidized metal-bearing fluids were introduced, and doubly so if the hydrocarbon fluid was contaminated by sour gas and contained appreciable amounts of hydrogen sulfide as is often the case with Mississippi Valley-type Pb–Zn deposits (Anderson, 2008; Huston et al., 2006). On encountering this reducing environment, a number of catalysed redox reactions ensued, during the course of which existing carbonate minerals were dissolved and metal sulfides precipitated in their place. Metal precipitation in other Pb–Zn deposits across the region, including Mount Isa, was likely driven by similar replacement reactions following upward transport of metalliferous fluids sourced from deeper levels of the basin. Fluids may initially have travelled up reactivated faults but on arrival at the ore formation site became trapped and migrated into their hangingwalls or footwall shortcut thrusts where they brought about dissolution of the host rock and its replacement by sulfides. This compares with current ore formation models where the mineralizing fluid was introduced along basin-bounding structures active at the time of basin formation (Huston et al., 2006; Large et al., 2005; McGoldrick et al., 2010; Southgate et al., 2000b) or along normal faults reactivated in the opposite sense during periods of transient crustal shortening in an overall extensional or sag setting (Betts et al., 2003; Kunzmann et al., 2019). Mineral exploration has accordingly often been directed towards the identification of structures and sedimentary sequences formed during the course of basin formation as opposed to those that may have formed during later basin inversion.
However, as is now evident from recent seismic interpretations of line 06GAM2 (Gibson et al., 2017, 2016), the carbonaceous rocks hosting Century belong to the syn-inversion fraction of basin fill and were deposited at a time of crustal shortening accompanying the Isan Orogeny. There is no evidence that this shortening was accompanied by reactivation of the basin-bounding structure. Instead, as with many normal faults, the Riversleigh Fault dipped too steeply to be easily reactivated and strain was taken up on a footwall shortcut thrust; it rather than the Riversleigh Fault would have served as the better fluid conduit. Interestingly, the Termite Range Fault has some of the same attributes as a footwall shortcut thrust and is widely believed (Broadbent et al., 1998; Yang and Radulescu, 2006) to have been the main fluid conduit for the Century deposit which lies either above or in a minor offshoot immediately adjacent to the master structure. No less importantly, mineralization is transgressive with respect to stratigraphy and occurred through replacement processes (Broadbent et al., 1998), consistent with the 1575 Ma age reported for this deposit (Carr et al., 2004). This is long after the start of crustal shortening and probable concomitant expulsion of hydrocarbons to higher stratigraphic levels where they would have pooled or been trapped in structures formed during inversion. It seems further likely, if the analogy with the petroleum system has any validity, that a significant amount of this metal-bearing fluid found its way into the same type of hangingwall structures that are so prospective of oil and gas in the younger inverted basins of the North Atlantic petroleum province. If this is indeed the case, then a change in exploration strategies for sediment-hosted Pb–Zn mineralization may be warranted where there is less focus on the identification of normal faults active at the time of basin formation towards increased targeting of potential structural traps located in either the footwalls or less proximal parts of their inverted hangingwall structures.
Importantly, owing to asymmetries inherited from the original basin architecture, the default position for the latter will be the same regions where basin fill attained maximum thickness and source rocks were sufficiently deeply buried to generate the requisite volumes of metalliferous fluids during basin inversion. Potential source rocks for Pb and Zn metal include the syn-rift component of basin fill which, in these regions, might be expected to include thick sequences of altered volcanic and immature sedimentary rocks as was reportedly the case for mineral deposits in the McArthur Basin and Leichhardt River fault trough (Cooke et al., 1998; Heinrich et al., 1995; Huston et al., 2016, 2006; Polito et al., 2006; Southgate et al., 2006; Champion et al., 2020). However, as revealed by the seismic data, substantial amounts of the Calvert and Leichhardt superbasins were removed through erosion during inversion so that neither the full complement of syn-rift rocks nor any vestige of a basin seal need be preserved in one or both basins (e.g. 17GA–SN1 and SN2). Basin seals and barriers to upward fluid flow are more likely to be found among the finer-grained carbonaceous shales and dolomitic siltstones making up the post-rift fraction of basin fill, but this is the very component most susceptible to uplift and erosion during the initial stages of basin inversion. Accordingly, the optimal site for the formation and preservation of Pb–Zn mineralization may be in basins whose inverted hangingwalls and related structures never lost all of their post-rift capping rocks and continued to evolve beneath succeeding layers of tectonically driven sedimentation. In either event, it is difficult to avoid the conclusion that basin inversion played an important, if not critical, role in the formation of a world-class Pb–Zn mineral province in northern Australia.
Uninterpreted seismic reflection images on which this paper is based are available online from the respective government data repositories: (Geoscience Australia eCat: http://pid.geoscience.gov.au/dataset/ga/69674, Maher, 2008; and http://pid.geoscience.gov.au/dataset/ga/116881, Holzschuh, 2018) and Queensland Geological Survey: https://qdexdata.dnrme.qld.gov.au/QDEXDataDownloadManager/Results?type=Seismic&id=95456,95541,91004 (Queensland Government, 2019).
GMG undertook most of the seismic interpretations, compiled the figures, and wrote the initial draft of the article; SE critically reviewed the seismic interpretations and initial draft of the paper, researched the implications of basin inversion on the petroleum system, and contributed written commentary on the latter that formed the foundations of a revised and expanded discussion section. Both authors participated in a PowerPoint discussion on basin inversion at GSQ in late 2019 that formed the basis for this paper.
The authors declare that they have no conflict of interest.
This article is part of the special issue “Inversion tectonics – 30 years later”. It is not associated with a conference.
For the invitation and opportunity to contribute to the special anniversary volume on basin inversion we thank the editors Jonas Kley (special issue) and Piotr Krzywiec (paper and special issue). For detailed and comprehensive reviews that greatly improved the text we are indebted to Alan Collins and Karen Connors. Thanks also to Teck Resources Australia and Pursuit Minerals for permission to publish our interpretation of the Punjaub Structure. Figure 2 was produced on our behalf by Ian Withnall, Geological Survey of Queensland.
This paper was edited by Piotr Krzywiec and reviewed by Karen Connors and Alan Collins.
Ahmad, M. and Munson, T. J.: Chapter 18: Lawn Hill Platform, in: Geology and mineral resources of the Northern Territory, Special Publication 5, edited by: Ahmad, M. and Munson, T. J., Northern Territory Geological Survey, Darwin, 2013.
Anderson, G. M.: The mixing hypothesis and the origin of Mississippi Valley-type deposits, Econ. Geol., 103, 1683–1690, 2008.
Andrews, S. J.: Stratigraphy and depositional setting of the upper McNamara Group, Lawn Hill region, northwest Queensland, Econ. Geol., 93, 1132–1152, https://doi.org/10.2113/gsecongeo.93.8.1132, 1998.
Baker, M. J., Crawford, A. J., and Withnall, I. W.: Geochemical, Sm-Nd isotopic characteristics and petrogenesis of Paleoproterozoic mafic rocks from the Georgetown Inlier, north Queensland: Implications for relationship with the Broken Hill and Mount Isa eastern succession, Precambrian Res., 177, 39–54, 2010.
Betts, P. G. and Giles, D.: The 1800–1100 Ma tectonic evolution of Australia, Precambrian Res., 144, 92–125, 2006.
Betts, P. G. and Lister, G. S.: Comparison of the “strike-slip” versus the “episodic rift-sag” models for the origin of the Isa Superbasin, Aust. J. Earth Sci., 48, 265–280, 2001.
Betts, P. G., Giles, D., and Lister, G. S.: Tectonic environment of shale-hosted massive sulphide Pb-Zn-Ag deposits of Proterozoic northeastern Australia, Econ. Geol., 98, 557–576, 2003.
Betts, P. G., Giles, D., and Lister, G. S.: Aeromagnetic patterns of half-graben and basin inversion: Implications for sediment-hosted massive sulfide Pb–Zn–Ag exploration, J. Struct. Geol., 26, 1137–1156, https://doi.org/10.1016/j.jsg.2003.11.020, 2004.
Betts, P. G., Giles, D., Mark, G., Lister, G. S., Goleby, B. R., and Ailleres, L.: Synthesis of the Proterozoic evolution of the Mount Isa inlier, Aust. J. Earth Sci., 53, 187–211, 2006.
Betts, P. G., Armit, R. J., Stewart, J., Aitken, A. R. A., Ailleres, L., Donchak, P., Hutton, L., Withnall, I., and Giles, D.: Australia and Nuna, Geol. Soc. Lond. Spec. Publ., 424, 47–81, https://doi.org/10.1144/sp424.2, 2016.
Bierlein, F. P., Black, L. P., Hergt, J., and Mark, G.: Evolution of pre-1.8 Ga basement rocks in the western Mt Isa Inlier, northeastern Australia–insights from Shrimp U-Pb dating and in-situ Lu-Hf analysis of zircons, Precambrian Res., 163, 159–173, 2008.
Bierlein, F. P., Maas, R., and Woodhead, J.: Pre-1.8 Ga tectono-magmatic evolution of the Kalkadoon–Leichhardt belt: Implications for the crustal architecture and metallogeny of the Mount Isa Inlier, northwest Queensland, Australia, Aust. J. Earth Sci., 58, 887–915, https://doi.org/10.1080/08120099.2011.571286, 2011.
Black, L. P. and McCulloch, M. T.: Isotopic evidence for the dependence of recurrent felsic magmatism on new crust formation: An example from the Georgetown region of northeastern Australia, Geochim. Cosmochim. Ac., 54, 183–196, 1990.
Black, L. P., Gregory, P., Withnall, I. W., and Bain, J. H. C.: U-Pb zircon age for the Etheridge Group, Georgetown region, north Queensland: Implications for relationship with the Broken Hill and Mt Isa sequences, Aust. J. Earth Sci., 45, 925–935, 1998.
Blaikie, T. N., Betts, P. G., Armit, R. J., and Ailleres, L.: The ca. 1740–1710 Ma Leichhardt Event: Inversion of a continental rift and revision of the tectonic evolution of the North Australian Craton, Precambrian Res., 292, 75–92, https://doi.org/10.1016/j.precamres.2017.02.003, 2017.
Blake, D. H.: Geology of the Mount Isa inlier and environs, Queensland and Northern Territory, BMR Bulletin, 225, 83 pp., 1987.
Bradshaw, B. E. and Scott, D. J.: Integrated basin analysis of the Isa Superbasin using seismic, well-log and geopotential data: An evaluation of the economic potential of the northern Lawn Hill Platform, Australian Geological Survey Organisation (now Geoscience Australia) AGSO Record 199/19 (Digital Version), Canberra, 1999.
Bradshaw, B. E., Lindsay, J. F., Krassay, A. A., and Wells, A. T.: Attenuated basin-margin sequence stratigraphy of the Palaeoproterozoic Calvert and Isa superbasins: The Fickling Group, southern Murphy Inlier, Queensland, Aust. J. Earth Sci., 47, 599–623, 2000.
Bradshaw, B. E., Orr, M. L., Bailey, A. H. E., Palu, T. J., and Hall, L. S.: Northern Lawn Hill Platform: Depth, structure and isochore mapping update, Geoscience Australia Record 2018/47, 75 pp., Geoscience Australia, Canberra, 2018.
Broadbent, G. C., Myers, R. E., and Wright, J. V.: Geology and origin of shale-hosted Zn-Pb-Ag mineralization at the Century deposit, northwest Queensland, Australia, Econ. Geol., 93, 1264–1294, https://doi.org/10.2113/gsecongeo.93.8.1264, 1998.
Carr, G. R., Denton, G. J., Parr, J., Sun, S.-S., Korsch, R. J., and Boden, S. B.: Lightning does strike twice; multiple ore events in major mineralised systems in northern Australia, in: Predictive mineral discovery under cover, extended abstracts, edited by: Muhling, J., Goldfarb, R., Vielreicher, N., Bierlein, F., Stumpfl, E., Groves, D. I., and Kenworthy, S., Centre for Global Metallogeny, The University of Western Australia, Perth, 2004.
Carr, L. K., Southby, C., Henson, P., Costelloe, R., Anderson, J. R., Jarrett, A. J. M., Carson, C. J., MacFarlane, S. K., Gorton, J., Hutton, L. J., Troup, A., Williams, B., Khider, K., Bailey, A. H. E., and Fomin, T.: Exploring for the future: South Nicholson Basin geological summary and seismic data interpretation, Record 2019/21, Geoscience Australia, Canberra, 2019.
Champion, D. C., Huston, D. L., Bastrakov, E. N., Siegel, C., Thorne, J., Gibson, G. M., and Hauser, J.: Alteration of mafic igneous rocks of the southern McArthur Basin: comparisons with the Mount Isa region and implications for basin-hosted base metal deposits, in: Exploring for the Future: extended abstracts, edited by: Czarnota, K., Roach, I. C., Abbott, S. T., Haynes, M. W., Kositcin, N., Ray, A., and Slatter, E., Geoscience Australia, Canberra, 1–6, https://doi.org/10.11636/134206, 2020.
Cooke, D. R., Bull, S. W., Donovan, S., and Rogers, J. R.: K-metasomatism and base metal depletion in volcanic rocks from the McArthur Basin, Northern Territory–implications for base metal mineralization, Econ. Geol., 93, 1237–1263, 1998.
Cooper, M. A., Collins, D., Ford, M., Murphy, F. X., and Trayner, P. M.: Structural style, shortening estimates and the thrust front of the Irish Variscides, Geol. Soc. Lond. Spec. Publ., 14, 167–175, https://doi.org/10.1144/gsl.sp.1984.014.01.16, 1984.
Cooper, M. A., Williams, G. D., de Graciansky, P. C., Murphy, R. W., Needham, T., de Paor, D., Stoneley, R., Todd, S. P., Turner, J. P., and Ziegler, P. A.: Inversion tectonics – a discussion, Geol. Soc. Lond. Spec. Publ., 44, 335–347, https://doi.org/10.1144/gsl.sp.1989.044.01.18, 1989.
Domagala, J., Southgate, P. N., McConachie, B. A., and Pidgeon, B. A.: Evolution of the Palaeoproterozoic Prize, Gun and lower Loretta supersequences of the Surprise Creek Formation and Mt Isa Group, Aust. J. Earth Sci., 47, 485–507, 2000.
Dooley, T. and McClay, K. R.: Analog modeling of pull-apart basins, AAPG Bulletin, 81, 1804–1826, 1997.
Duncan, R. J., Stein, H. J., Evans, K. A., Hitzman, M. W., Nelson, E. P., and Kirwin, D. J.: A new geochronological framework for mineralization and alteration in the Selwyn-Mount Dore corridor, eastern fold belt, Mount Isa Inlier, Australia: Genetic implications for iron oxide copper-gold deposits, Econ. Geol., 106, 169–192, https://doi.org/10.2113/econgeo.106.2.169, 2011.
Foster, D. R. W. and Austin, J. R.: The 1800–1610 Ma stratigraphic and magmatic history of the eastern succession, Mount Isa Inlier, and correlations with adjacent Paleoproterozoic terranes, Precambrian Res., 163, 7–30, https://doi.org/10.1016/j.precamres.2007.08.010, 2008.
Foster, D. R. W. and Rubenach, M.: Isograd patterns and regional low-pressure- high-temperature metamorphism of pelitic, mafic and calc-silicate rocks along an east-west section through the Mount Isa Inlier, Aust. J. Earth Sci., 53, 167–186, 2006.
Frogtech Geoscience: North West Queensland SEEBASE® Study and GIS, Queensland Geological Record 2018/03, Brisbane, 2018.
Gibson, G. M., Rubenach, M. J., Neumann, N. L., Southgate, P. N., and Hutton, L. J.: Syn- and post-extensional tectonic activity in the Palaeoproterozoic sequences of Broken Hill and Mount Isa and its bearing on reconstructions of Rodinia, Precambrian Res., 166, 350–369, https://doi.org/10.1016/j.precamres.2007.05.005, 2008.
Gibson, G. M., Henson, P. A., Neumann, N. L., Southgate, P. N., and Hutton, L. J.: Paleoproterozoic-earliest Mesoproterozoic basin evolution in the Mount Isa region, northern Australia and implications for reconstructions of the Nuna and Rodinia supercontinents, Episodes, 35, 131–141, 2012.
Gibson, G. M., Meixner, A. J., Withnall, I. W., Korsch, R. J., Hutton, L. J., Jones, L. E. A., Holzschuh, J., Costelloe, R. D., Henson, P. A., and Saygin, E.: Basin architecture and evolution in the Mount Isa mineral province, northern Australia: Constraints from deep seismic reflection profiling and implications for ore genesis, Ore Geol. Rev., 76, 414–441, https://doi.org/10.1016/j.oregeorev.2015.07.013, 2016.
Gibson, G. M., Hutton, L. J., and Holzschuh, J.: Basin inversion and supercontinent assembly as drivers of sediment-hosted Pb–Zn mineralization in the Mount Isa region, northern Australia, J. Geol. Soc., 174, 773–786, https://doi.org/10.1144/jgs2016-105, 2017.
Gibson, G. M., Champion, D. C., Withnall, I. W., Neumann, N. L., and Hutton, L. J.: Assembly and breakup of the Nuna supercontinent: Geodynamic constraints from 1800 to 1600 Ma sedimentary basins and basaltic magmatism in northern Australia, Precambrian Res., 313, 148–169, https://doi.org/10.1016/j.precamres.2018.05.013, 2018.
Gibson, G. M., Champion, D. C., Huston, D. L., and Withnall, I. W.: Orogenesis in Paleo-Mesoproterozoic eastern Australia: A response to arc-continent and continent-continent collision during assembly of the Nuna supercontinent, Tectonics, 39, e2019TC005717, https://doi.org/10.1029/2019tc005717, 2020.
Giles, D., Betts, P. G., and Lister, G. S.: Far-field continental back-arc setting for the 1.8–1.67 Ga basins of north-east Australia, Geology, 30, 823–826, 2002.
Giles, D., Betts, P. G., and Lister, G. S.: 1.8–1.5 Ga links between the North and South Australian cratons and the early–middle Proterozoic configuration of Australia, Tectonophysics, 380, 27–41, https://doi.org/10.1016/j.tecto.2003.11.010, 2004.
Giles, D., Betts, P. G., Ailleres, L., Hulscher, B., Hough, M., and Lister, G. S.: Evolution of the Isan Orogeny at the southeastern margin of the Mt Isa Inlier, Aust. J. Earth Sci., 53, 91–108, 2006.
Glikson, A. Y., Derrick, G. M., Wilson, I. H., and Hill, R. M.: Tectonic evolution and crustal setting of the middle Proterozoic Leichhardt River Fault Trough, Mount Isa region, northwest Queensland, BMR Journal of Australian Geology and Geophysics, 1, 115–129, 1976.
Glikson, M., Golding, S. D., and Southgate, P. N.: Thermal evolution of the ore-hosting Isa Superbasin: Central and northern Lawn Hill Platform, Econ. Geol., 101, 1211–1229, https://doi.org/10.2113/gsecongeo.101.6.1211, 2006.
Golding, S. D., Uysal, I. T., Glikson, M., Baublys, K. A., and Southgate, P. N.: Timing and chemistry of fluid-flow events in the Lawn Hill Platform, northern Australia, Econ. Geol., 101, 1231–1250, https://doi.org/10.2113/gsecongeo.101.6.1231, 2006.
Gorton, J. and Troup, A.: Petroleum systems of the Proterozoic in northwest Queensland and a description of various play types, APPEA Journal, 58, 311–320, https://doi.org/10.1071/AJ17115, 2018.
Hayward, A. B. and Graham, R. H.: Some geometrical characteristics of inversion, Geol. Soc. Lond. Spec. Publ., 44, 17–39, https://doi.org/10.1144/gsl.sp.1989.044.01.03, 1989.
Heinrich, C. A., Bain, J. H. C., Mernagh, T. P., Wyborn, L. A. I., Andrew, A. S., and Waring, C. L.: Fluid and mass transfer during metabasalt alteration and copper mineralisation at Mount Isa, Australia, Econ. Geol., 90, 705–730, 1995.
Hinman, M.: Base metal mineralisation at McArthur River: Structure and kinematics of the HYC-Cooley Zone, Australian Geological Survey Organisation Record 1995/5, Canberra, 41 pp., 1995.
Holcombe, R. J., Pearson, P. J., and Oliver, N. H. S.: Geometry of a middle Proterozoic extensional decollement in north-eastern Australia, Tectonophysics, 191, 255–274, 1991.
Holzschuh, J.: L210 South Nicholson Deep Crustal Seismic Reflection Survey, NT and QLD, 2017, Geoscience Australia, Canberra, http://pid.geoscience.gov.au/dataset/ga/116881 (last access: 2019), 2018.
Huston, D. L., Stevens, B., Southgate, P. N., Muhling, P., and Wyborn, L.: Australian Zn-Pb-Ag ore-forming systems: A review and analysis, Econ. Geol., 101, 1117–1157, https://doi.org/10.2113/gsecongeo.101.6.1117, 2006.
Huston, D. L., Mernagh, T. P., Hagemann, S. G., Doublier, M. P., Fiorentini, M., Champion, D. C., Lynton Jaques, A., Czarnota, K., Cayley, R., Skirrow, R., and Bastrakov, E.: Tectono-metallogenic systems – the place of mineral systems within tectonic evolution, with an emphasis on Australian examples, Ore Geol. Rev., 76, 168–210, https://doi.org/10.1016/j.oregeorev.2015.09.005, 2016.
Hutton, L. J. and Sweet, I. P.: Geological evolution, tectonic style and economic potential of the Lawn Hill Platform cover, northwest Queensland, BMR Journal of Australian Geology and Geophysics, 7, 125–134, 1982.
Jackson, M. J., Powell, T. G., Summons, R. E., and Sweet, I. P.: Hydrocarbon shows and petroleum source rocks in sediments as old as 1.7×109 years, Nature, 322, 727–729, https://doi.org/10.1038/322727a0, 1986.
Jackson, M. J., Scott, D. L., and Rawlings, D. J.: Stratigraphic framework for the Leichhardt and Calvert superbasins: Review and correlations of the pre-1700 Ma successions between Mt Isa and McArthur River, Aust. J. Earth Sci., 47, 381–403, https://doi.org/10.1046/j.1440-0952.2000.00789.x, 2000.
Jarrett, A. J. M., Cox, G. M., Southby, C., Hong, Z., Palatty, P., Carr, L., and Henson, P.: Source rock geochemistry of the McArthur Basin, northern Australia: Rock-eval pyrolysis data release. Record 2018/24. Geoscience Australia, Canberra, https://doi.org/10.11636/Record.2018.024, 2018.
Kesler, S. E., Jones, H. D., Furman, F. C., Sassen, R., Anderson, W. H., and Kyle, J. R.: Role of crude oil in the genesis of Mississippi Valley-type deposits: Evidence from the Cincinnati Arch, Geology, 22, 609–612, https://doi.org/10.1130/0091-7613(1994)022<0609:rocoit>2.3.co;2, 1994.
Korsch, R. J., Huston, D. L., Henderson, R. A., Blewett, R. S., Withnall, I. W., Fergusson, C. L., Collins, W. J., Saygin, E., Kositcin, N., Meixner, A. J., Chopping, R., Henson, P. A., Champion, D. C., Hutton, L. J., Wormald, R., Holzschuh, J., and Costelloe, R. D.: Crustal architecture and geodynamics of north Queensland, Australia: Insights from deep seismic reflection profiling, Tectonophysics, 572–573, 76–99, https://doi.org/10.1016/j.tecto.2012.02.022, 2012.
Kositcin, N. and Carson, C. J.: New shrimp U–Pb zircon ages from the South Nicholson and Carrara Range region, Northern Territory, Record 2019/09, Geoscience Australia, Canberra, 2019.
Krassay, A. A., Bradshaw, B. E., Domagala, J., and Jackson, M. J.: Siliciclastic shoreline to growth-faulted, turbiditic sub-basins: The Proterozoic River Supersequence of the upper McNamara Group on the Lawn Hill Platform, northern Australia, Aust. J. Earth Sci., 47, 533–562, 2000a.
Krassay, A. A., Domagala, J., Bradshaw, B. E., and Southgate, P. N.: Lowstand ramps, fans and deep-water Palaeoproterozoic and Mesoproterozoic facies of the Lawn Hill Platform: The Term, Lawn, Wide and Doom supersequences of the Isa Superbasin, northern Australia, Aust. J. Earth Sci., 47, 563–597, 2000b.
Kunzmann, M., Schmid, S., Blaikie, T. N., and Halverson, G. P.: Facies analysis, sequence stratigraphy, and carbon isotope chemostratigraphy of a classic Zn-Pb host succession: The Proterozoic middle McArthur Group, McArthur Basin, Australia, Ore Geol. Rev., 106, 150–175, https://doi.org/10.1016/j.oregeorev.2019.01.011, 2019.
Large, R. R., Bull, S. W., McGoldrick, P. J., Walters, S., Derrick, G. M., and Carr, G. R.: Stratiform and strata-bound Zn-Pb-Ag deposits in Proterozoic sedimentary basins, northern Australia, Economic Geology 100th Anniversary Volume, Society of Economic Geologists, Colorado, USA, 931–963, 2005.
Leach, D. L., Bradley, D., Lewchuk, M. T., Symons, D. T., de Marsily, G., and Brannon, J.: Mississippi Valley-type lead–zinc deposits through geological time: Implications from recent age-dating research, Miner. Deposita, 36, 711–740, https://doi.org/10.1007/s001260100208, 2001.
Leach, D. L., Bradley, D. C., Huston, D., Pisarevsky, S. A., Taylor, R. D., and Gardoll, S. J.: Sediment-hosted lead-zinc deposits in earth history, Econ. Geol., 105, 593–625, https://doi.org/10.2113/gsecongeo.105.3.593, 2010.
Lowell, J. D.: Mechanics of basin inversion from worldwide examples, Geol. Soc. Lond. Spec. Publ., 88, 39–57, https://doi.org/10.1144/gsl.sp.1995.088.01.04, 1995.
Maher, J. L.: L180 Mt Isa Deep Crustal Seismic Survey, QLD, 2006. Stacked and migrated data and images for lines 06GA-M1 to 06GA-M6, Geoscience Australia, Canberra, available at: http://pid.geoscience.gov.au/dataset/ga/69674 (last access: 2019), 2008.
Martínez, F., Arriagada, C., Mpodozis, C., and Peña, M.: The Lautaro Basin: A record of inversion tectonics in northern Chile, Andean Geol., 39, 258–278, 2012.
McClay, K. R.: The geometries and kinematics of inverted fault systems: A review of analogue model studies, Geol. Soc. Lond. Spec. Publ., 88, 97–118, https://doi.org/10.1144/gsl.sp.1995.088.01.07, 1995.
McClay, K. R. and White, M. J.: Analogue modelling of orthogonal and oblique rifting, Mar. Petrol. Geol., 12, 137–151, 1995.
McClay, K. R., Dooley, T., Whitehouse, P., and Mills, M.: 4-D evolution of rift systems: Insights from scaled physical models, AAPG Bulletin, 86, 935–959, https://doi.org/10.1306/61eedbf2-173e-11d7-8645000102c1865d, 2002.
McConachie, B. A. and Dunster, J. N.: Sequence stratigraphy of the Bowthorn block in the northern Mount Isa basin, Australia: Implications for the base-metal mineralization process, Geology, 24, 155–158, https://doi.org/10.1130/0091-7613(1996)024<0155:ssotbb>2.3.co;2, 1996.
McConachie, B. A., Barlow, M. G., Dunster, J. N., Meaney, R. A., and Schaap, A. D.: The Mount Isa basin-definition, structure and petroleum geology, APEA Journal, 33, 237–257, 1993.
McGoldrick, P., Winefield, P., Bull, S., Selley, D., and Scott, R. J.: Sequences, synsedimentary structures, and sub-basins: The where and when of sedex zinc systems in the southern McArthur Basin, Australia, Soc. Eco. Geo. Spc. Pub., 15, 367–389, 2010.
Neumann, N. L., Southgate, P. N., Gibson, G. M., and McIntyre, A.: New shrimp geochronology for the westwern fold belt of the Mount Isa Inlier: Developing a 1800–1650 Ma event framework, Aust. J. Earth Sci., 53, 1023–1039, 2006.
Neumann, N. L., Gibson, G. M., and Southgate, P. N.: New shrimp age constraints on the timing and duration of magmatism and sedimentation in the Mary Kathleen Fold Belt, Mt Isa Inlier, Aust. J. Earth Sci., 56, 965–983, 2009.
O'Dea, M. G., Lister, G. S., Betts, P. G., and Pound, K. S.: A shortened intraplate rift system in the Proterozoic Mount Isa terrane, N. W. Queensland, Australia, Tectonics, 16, 425–441, 1997a.
O'Dea, M. G., Lister, G. S., MacCready, T., Betts, P. G., Oliver, N. H. S., Pound, K. S., Huang, W., Valenta, R. K., Oliver, N. H. S., and Valenta, R. K.: Geodynamic evolution of the Proterozoic Mount Isa terrain, Geol. Soc. Lond. Spec. Publ., 121, 99–122, https://doi.org/10.1144/gsl.sp.1997.121.01.05, 1997b.
Page, R. W. and Sun, S. S.: Aspects of geochronology and crustal evolution in the eastern fold belt, Mt Isa Inlier, Aust. J. Earth Sci., 45, 343–361, https://doi.org/10.1080/08120099808728396, 1998.
Page, R. W., Jackson, M. J., and Krassay, A. A.: Constraining sequence stratigraphy in north Australian basins: Shrimp U-Pb zircon geochronology between Mt Isa and McArthur River, Aust. J. Earth Sci., 47, 431–459, 2000.
Pearson, P. J., Holcombe, R. J., and Page, R. W.: Synkinematic emplacement of the middle Proterozoic Wonga Batholith into a midcrustal shear zone, Mount Isa Inlier, Queensland, Australia, in: Detailed studies of the Mount Isa Inlier: Australian Geological Survey Organisation Bulletin 243, edited by: Stewart, A. J. and Blake, D. H., Canberra, 289–328, 1991.
Polito, P. A., Kyser, T. K., Golding, S., D., and Southgate, P. N.: Zinc deposits and related mineralization of the Burketown mineral field, including the world-class Century deposit, northern Australia: Fluid inclusion and stable isotope evidence for basin fluid sources, Econ. Geol., 101, 1251–1273, https://doi.org/10.2113/gsecongeo.101.6.1251, 2006.
Pollard, P. and McNaughton, N. J.: U/Pb geochronology and Sm/Nd isotope characterization of Proterozoic intrusive rocks in the Cloncurry district, Mount Isa Inlier, Australia, Amira P438 Cloncurry base metals and gold final report: Section 4, Australian Mineral Industries Research Association, Melbourne, 19 pp., 1997.
Pollard, P. J., Mark, G., and Mitchell, L. C.: Geochemistry of post-1540 Ma granites spatially associated with regional sodic-calcic alteration and Cu-Au-Co mineralisation, Cloncurry district, northwest Queensland, Econ. Geol., 93, 1330–1344, 1998.
Pourteau, A., Smit, M. A., Li, Z.-X., Collins, W. J., Nordsvan, A. R., Volante, S., and Li, J.: 1.6 Ga crustal thickening along the final Nuna suture, Geology, 46, 959–962, https://doi.org/10.1130/G45198.1, 2018.
Pursuit Minerals: Pursuit Minerals Limited lists on ASX and immediately commences Bluebush drilling program, available at: http://pursuitminerals.Com.Au/wp-content/uploads/2017/09/pur-lists-on-ASX-and-commences-Bluebush-drilling-program.Pdf (last access: 2019), 2017.
Queensland Government: DNRME, available at: https://qdexdata.dnrme.qld.gov.au/QDEXDataDownloadManager/Results?type=Seismic&id=95456,95541,91004, last access: 2019.
Rawlings, D. J., Sweet, I. P., and Kruse, P. D.: Mount Drummond, Northern Territory. 1 : 250 000 geological map series explanatory notes, SE 53-12, Northern Territory Geological Survey, Darwin, 2008.
Rohrlach, B. D., Fu, M., and Clarke, J. D. A.: Geological setting, paragenesis and fluid history of the Walford Creek Zn-Pb-Cu-Ag prospect, Mt Isa basin, Australia, Aust. J. Earth Sci., 45, 63–81, https://doi.org/10.1080/08120099808728367, 1998.
Rubenach, M. J., Foster, D. R. W., Evins, P. M., Blake, K. L., and Fanning, C. M.: Age constraints on the tectonothermal evolution of the Selwyn zone, eastern fold belt, Mount Isa Inlier, Precambrian Res., 163, 81–107, 2008.
Scott, D. L., Bradshaw, B. E., and Tarlowski, C. Z.: The tectonostratigraphic history of the Proterozoic northern Lawn Hill Platform, Australia: An integrated intracontinental basin analysis, Tectonophysics, 300, 329–358, https://doi.org/10.1016/S0040-1951(98)00253-4, 1998.
Scott, D. L., Rawlings, D. J., Page, R. W., Tarlowski, C. Z., Idnurm, M., Jackson, M. J., and Southgate, P. N.: Basement framework and geodynamic evolution of the Palaeoproterozoic superbasins of north-central Australia: An integrated review of geochemical, geochronological and geophysical data, Aust. J. Earth Sci., 47, 341–380, 2000.
Southgate, P. N., Bradshaw, B. E., Domagala, J., Jackson, M. J., Idnurm, M., Krassay, A. A., Page, R. W., Sami, T. T., Scott, D. L., Lindsay, J. F., McConachie, B. A., and Tarlowski, C.: Chronostratigraphic basin framework for Palaeoproterozoic rocks (1730–1575 Ma) in northern Australia and implications for base-metal mineralisation, Aust. J. Earth Sci., 47, 461–483, 2000a.
Southgate, P. N., Scott, D. L., Sami, T. T., Domagala, J., Jackson, M. J., James, N. P., and Kyser, T. K.: Basin shape and sediment architecture in the Gun Supersequence: A strike-slip model for Pb-Zn-Ag ore genesis at Mt Isa, Aust. J. Earth Sci., 47, 509–531, 2000b.
Southgate, P. N., Kyser, T. K., Scott, D. L., Large, R. R., Golding, S. D., and Polito, P. A.: A basin system and fluid-flow analysis of the Zn-Pb-Ag Mount Isa-type deposits of northern Australia: Identifying metal source, basinal brine reservoirs, times of fluid expulsion, and organic matter reactions, Econ. Geol., 101, 1103–1115, https://doi.org/10.2113/gsecongeo.101.6.1103, 2006.
Southgate, P. N., Neumann, N. L., and Gibson, G. M.: Depositional systems in the Mt Isa inlier from 1800 Ma to 1640 Ma: Implications for Zn–Pb–Ag mineralisation, Aust. J. Earth Sci., 60, 157-173, 2013.
Sweet, I. P.: Carrara Range region NT Geological Map Commentary, Record 6460, Geoscience Australia, Canberra, 1984.
Sweet, I. P.: The geology of the South Nicholson Group, northwest Queensland, Queensland Geological Record 2017/07, Queensland Geological Survey, Brisbane, 2017.
Thomas, D. W. and Coward, M. P.: Late Jurassic-early Cretaceous inversion of the northern east Shetland Basin, northern North Sea, Geol. Soc. Lond. Spec. Publ., 88, 275–306, https://doi.org/10.1144/gsl.sp.1995.088.01.16, 1995.
Turner, J. P. and Williams, G. A.: Sedimentary basin inversion and intra-plate shortening, Earth-Sci. Rev., 65, 277–304, https://doi.org/10.1016/j.earscirev.2003.10.002, 2004.
Withnall, I. W.: Geochemistry and tectonic significance of Proterozoic mafic rocks from the Georgetown Inlier, north Queensland, BMR J. Aust. Geol. Geop., 9, 339–351, 1985.
Withnall, I. W. and Hutton, L. J.: Chapter 2: North Australian Craton, in: Geology of Queensland, edited by: Jell, P. A., Geological Survey of Queensland, Brisbane, 23–112, 2013.
Yang, B., Collins, A. S., Cox, G. M., Jarrett, A. J. M., Denyszyn, S., Blades, M. L., Farkaš, J., and Glorie, S.: Using Mesoproterozoic sedimentary geochemistry to reconstruct basin tectonic geography and link organic carbon productivity to nutrient flux from a northern Australian large igneous province, Basin Res., https://doi.org/10.1111/bre.12450, online first, 2020.
Yang, J. and Radulescu, M.: Paleo-fluid flow and heat transport at 1575 Ma over an E–W section in the northern Lawn Hill Platform, Australia: Theoretical results from finite element modeling, J. Geochem. Explor., 89, 445–449, https://doi.org/10.1016/j.gexplo.2005.11.079, 2006.
- Abstract
- Introduction
- Regional geology and basin-forming events of northern Australia
- Seismic record of Paleo–Mesoproterozoic basin formation and inversion in northern Australia
- Discussion
- Data availability
- Author contributions
- Competing interests
- Special issue statement
- Acknowledgements
- Review statement
- References
- Abstract
- Introduction
- Regional geology and basin-forming events of northern Australia
- Seismic record of Paleo–Mesoproterozoic basin formation and inversion in northern Australia
- Discussion
- Data availability
- Author contributions
- Competing interests
- Special issue statement
- Acknowledgements
- Review statement
- References