the Creative Commons Attribution 4.0 License.
the Creative Commons Attribution 4.0 License.
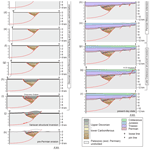
Together but separate: decoupled Variscan (late Carboniferous) and Alpine (Late Cretaceous–Paleogene) inversion tectonics in NW Poland
Piotr Krzywiec
Mateusz Kufrasa
Paweł Poprawa
Stanisław Mazur
Małgorzata Koperska
Piotr Ślemp
In Europe, formation of the Palaeozoic Variscan orogenic belt, and the Mesozoic–Cenozoic Alpine–Carpathian orogenic belt led to a widespread inversion events within forelands of both orogenic domains. We used legacy 2-D seismic data together with the newly acquired 3-D seismic data that, for the first time, precisely imaged sub-Zechstein (i.e. sub-evaporitic) upper Palaeozoic successions in NW Poland in order to develop a quantitative, balanced 2-D model of the late Palaeozoic–recent evolution of this area, characterised by a complex pattern of repeated extension and inversion. Four main tectonic phases have been determined: (1) Late Devonian–early Carboniferous extension and subsidence possibly related to extensional reactivation of Caledonian thrusts, (2) late Carboniferous inversion caused by the Variscan orogeny, (3) Permo-Mesozoic subsidence related to the development of the Polish Basin and (4) its Late Cretaceous–Paleogene inversion. Variscan and Alpine structures form a superimposed multilayer inversion system, mechanically decoupled by the Zechstein evaporites.
- Article
(14226 KB) - Full-text XML
- BibTeX
- EndNote
Inversion tectonics has been intensely studied since the early 1980s, when a fully developed concept of sedimentary basin inversion, exemplified by a tectonic graben bounded by deeply rooted normal faults subsequently reactivated as reverse faults or thrusts, was formulated (Glennie and Boegner, 1981; Bally, 1984). During this period, numerous papers and two dedicated volumes were published in which the geometry and evolution of inversion structures in different geodynamic settings were discussed (Cooper and Williams, 1989; Buchanan and Buchanan, 1995). Published case studies, documented by surface and/or subsurface data and supported by results of analogue and numerical modelling studies, dealt with the geometry of inversion systems, the role of differential lithologies in the formation of inversion structures, the evolution of fracture systems and syn-inversion sedimentation (e.g. Bonini et al., 2012; Buiter and Pfiffner, 2003; Buiter et al., 2009; Cloetingh et al., 2008; Dooley and Hudec, 2020; Henk and Nemčok, 2008; Jagger and McClay, 2018; McClay, 1995; Mitra and Islam, 1994; Panien et al., 2005, 2006).
Thanks to all this work, the scene for inversion tectonics seems to be fairly well set; there are, however, still some aspects that require further clarification. They include crucial topics of inversion tectonics such as definition of inversion structures and their key characteristics. In fact, inversion structures come in various shapes and forms, and, despite all the studies briefly mentioned above, inversion tectonics could be quite differently understood. To some, it might be regarded as a very general process that leads to reduction of the accommodation space and, eventually, cessation, i.e. inversion, of the entire sedimentary basin. The process that reduces tectonic subsidence, eventually bringing it to a halt and causing uplifts, might include reversal of basement normal faults that controlled localised tectonic subsidence as proposed in the classic model by Bally (1984). However, inversion tectonics could also involve other, sometimes very different processes such as salt movements, lithospheric buckling or isostatic adjustments (cf. Dewey, 1989; see also Kley, 2018; Krzywiec et al., 2018). Another, more “conservative” approach to inversion tectonics relies on the classic model by Bally (1984) that assumes (1) formation of tectonic graben bounded by normal fault(s) during extension and (2) compressional reactivation of graben-bounding fault(s) and uplift of sedimentary infill deposited during extension (cf. also Cooper et al., 1989; Cooper and Warren, 2010, 2020; Tari et al., 2020; Williams et al., 1989; Fig. 1). In this model, a master normal fault is deeply rooted in the crystalline basement so its reactivation under regional compression could be regarded as “thick-skinned inversion tectonics” (cf. Brun and Nalpas, 1996). An extensional phase is documented by divergent pattern of the growth strata that thicken towards the master fault. During inversion, formation of growth strata, characterised by local thinning, is focused above inversion anticlines that develop above the tip of inverted master fault (Fig. 1a). Well-documented examples of such deeply rooted inversion structures include the Precambrian Calvert and Isa basins from NE Australia (Gibson and Edwards, 2020), the Devonian–Carboniferous Dnipro–Donetsk Basin (Maystrenko et al., 2003), the Triassic–Jurassic Lautaro Basin in northern Chile (Martínez et al., 2012) or the Paleogene Song Hong and Beibuwan basins in northern Vietnam (Fyhn et al., 2018).
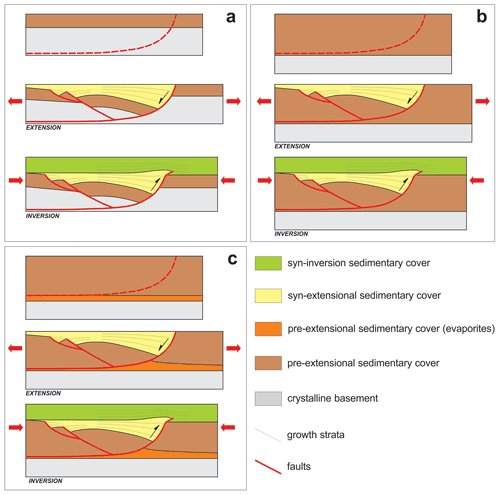
Figure 1(a) Classic thick-skinned model of inversion of half-graben with master listric fault rooted within the crystalline basement, based on Bally (1984); see also Cooper and Warren (2010, 2020) and Tari et al. (2020). (b) Thin-skinned model of inversion of half-graben with master listric fault rooted within the thick pre-extensional sedimentary cover. (c) Thin-skinned model of inversion of half-graben with master listric fault detached above pre-extensional evaporites. Note that all three models, despite their different structural characteristics at depth, have the same shallower tectono-sedimentary expression with identical key elements of the inverted extensional system such as inversion anticline and syn-inversion growth strata.
There are, however, numerous departures from this classic model of inversion tectonics. The most important difference is the location of main basal detachment that can develop not within the crystalline basement, as proposed by Bally (1984), but within the pre-extensional sedimentary cover (Fig. 1b and c). A common situation in which such a location of the main detachment of an extensional–inversion system could be encountered is related to foreland thin-skinned fold-and-thrust belts, where major thrusts could be first reactivated as normal faults and then inverted due to regional compression (e.g. Di Domenica et al., 2014; Tavarnelli, 1999; Withjack et al., 2010). Thin-skinned rather than thick-skinned character of inversion tectonics is in this case determined by a thin-skinned nature of the fold-and-thrust belt that undergoes reactivation. It is worth noting here that extensional reactivation of thrusts is often referred to as a “negative inversion”, in contrast to “positive inversion” associated with reverse reactivation of normal faults that was briefly described above (e.g. Chadwick and Smith, 1988; Del Ventisette et al., 2021; Krantz, 1991; Tari et al., 2021; Tortorici et al., 2019). In this case, however, “inversion” seems to be used as an equivalent of “extensional reactivation”, which is a much broader term that does not fully comply with the original concept of “inversion tectonics” coined by Bally (1984) (see also Holdsworth et al., 1997). Bally's original model was not focused on inversion, i.e. reactivation, of basin-bounding faults but on inversion, i.e. partial or full destruction, of a sedimentary basin. Destruction of a sedimentary basin (half-graben) in Bally's model is genetically linked to reactivation (reverse in this case) of a basin-bounding fault(s), but fault reactivation is a secondary process here, while the main emphasis is on demise of a half-graben formed during the extensional phase (Bally, 1984). On the other hand, negative inversion might be related to formation, not destruction, of a new basin that develops above extensionally reactivated thrust (e.g. Babaahmadi et al., 2018; Constenius, 1982, 1996; Deng et al., 2021; Powell and Williams, 1989; Tari et al., 2021; Velasco et al., 2010). Taking this into account, it seems appropriate to delimit usage of the term “inversion tectonics” to “positive inversion” as originally proposed by Bally (1984), and to abandon the term “negative inversion” that in fact is related to a rather different tectonic scenario. At the end of the day, however, this is all a matter of terminology and definitions, and general consensus (or lack thereof) around them. Different opinions on that issue have been already expressed; for example, Cooper and Warren (2020) in their recent chapter provided a short overview of various opinions on the term “negative inversion” and explicitly stated that it should be discarded. On the other hand, there are recently published important papers in which the term “negative inversion” has been successfully used to describe regional tectonic evolution of particular regions and formation of various structures within the Earth's crust (e.g. Tari et al., 2021; Connors and Houseknecht, 2022). In this paper, we follow our line of reasoning described above and report inferred extensional reactivation (not negative inversion) of Caledonian thrusts in northern Poland, the formation of upper Palaeozoic half-grabens and their subsequent inversion (see below).
The classic and relatively simple thin-skinned inversion scenario shown in Fig. 1b could be further complicated by lithological variations of the sedimentary infill formed prior to onset of inversion, i.e. prior to, during and after extension. The most obvious example of influence exerted by lithology on inversion tectonics is the presence of ductile evaporites that leads to partial or full mechanical decoupling and formation of sub- and supra-salt/evaporitic structures, often of different geometries and kinematics (e.g. Brun and Nalpas, 1996; Jackson and Larsen, 2008; Jackson et al., 2013; Dooley and Hudec, 2020; Hansen et al., 2021). A model of thin-skinned inversion system detached above evaporites is shown in Fig. 1c. One important conclusion might be drawn from comparison of “classic” thick-skinned inversion system (Fig. 1a) and two thin-skinned inversion systems, one without evaporites (Fig. 1b) and one with evaporites (Fig. 1c) is that despite their fundamentally different structural characteristics at depth, they all have the same shallower tectono-sedimentary expression with identical key elements of the inverted extensional system such as inversion anticline and syn-inversion growth strata. Taking this into account, it could be postulated that the “classic” inversion scenario of extensional graben (sedimentary basin) should not be restricted to basins with their bounding faults rooted within the crystalline basement and could equally well also be applied to thin-skinned systems, either developed above ductile evaporites or simply located above thick pre-extensional sedimentary cover that prevented faulting deeply rooted within the crystalline basement during extension and subsequent inversion (see also Cooper and Warren, 2020).
In Europe, formation of the Palaeozoic Variscan orogenic belt, and then the Mesozoic–Cenozoic Alpine–Carpathian orogenic belt, led to a widespread inversion events within forelands of both orogenic domains. Variscan (i.e. late Carboniferous) inversion is well documented in areas where either suitable outcrops of deformed Palaeozoic rocks are present or deeper seismic imaging is not hampered by a thick upper Permian (Zechstein) evaporitic cover, for example, the southern UK, Belgium or northern Germany (e.g. Chadwick and Evans, 2005; Pharaoh et al., 2020; Deckers and Rombaut, 2021; von Hartmann, 2003). On the other hand, Alpine (i.e. Late Cretaceous–Paleogene) inversion is well documented by seismic reflection data that image usually complex supra-salt/supra-evaporitic thin-skinned structures that are, however, characterised by not-always-clear relationships to the sub-salt/sub-evaporitic deformations (e.g. Chadwick and Evans, 2005; Mazur et al., 2005; Krzywiec, 2006b).
In this paper, we analyse complex inversion tectonics in NW Poland, where the superimposed effects of Variscan and Alpine foreland compression led to the formation of a multilayer decoupled inversion system. Our analysis is partly based on a newly acquired high-quality 3-D seismic data that provided unique, so-far-unavailable insight into the pre-Zechstein sedimentary cover. Geometry, kinematics and causal links between consecutive phases of extension/subsidence and compression/inversion are discussed in the context of the regional Palaeozoic and Mesozoic evolution of central and eastern Europe.
The study area in NW Poland (Figs. 2–5) is located in region where crystalline basement is buried to depths exceeding 11 km, as documented by deep seismic refraction data and gravity-magnetic modelling studies (Grad et al., 2002; Guterch et al., 1999, 2010; Guterch and Grad, 2006; Grad and Polkowski, 2016; Mazur et al., 2021). This is also compatible with the results of deep seismic reflection surveying in the area adjacent to the east that documented the Caledonian orogenic front thrust over the undeformed lower Palaeozoic foreland and underlain by a lower plate that gradually descends towards the SW to a depth of at least 10 km (Krzywiec et al., 2014; cf. also Lazauskienė et al., 2002; Mazur et al., 2018; Poprawa, 2019; Poprawa et al., 2020, 1999). Structures analysed in this paper evolved above the frontal part of this deeply buried Caledonian thin-skinned orogenic belt (Mazur et al., 2016), below which the Precambrian suture of the Teisseyre–Tornquist Zone is located (Mazur et al., 2015).
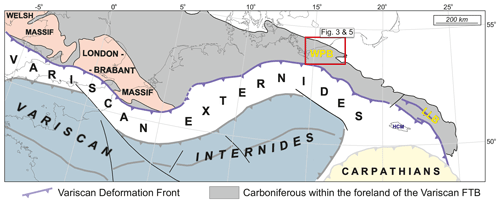
Figure 2Regional map showing main elements of the Variscan orogenic belt and its foreland between the UK/France and Poland/Ukraine (adopted from Krzywiec and Kufrasa, submitted, compiled after Mazur et al., 2020; Narkiewicz, 2007, 2020; Opluštil and Cleal, 2007; Ziegler, 1990; Martínez Catalan et al., 2020). Grey area: present-day post-erosional extent of the Carboniferous basin. WPB: western Pomeranian Basin, LLB: Lublin–Lviv Basin. Variscan orogenic front (thick dark violet line) is shown as a foreland-verging thrust but it should be kept in mind that this is regional generalisation meant to illustrate general vergence of the entire thrust belt and that along that front also backthrusting and wedging could be observed. HCM: Holy Cross Mountains.
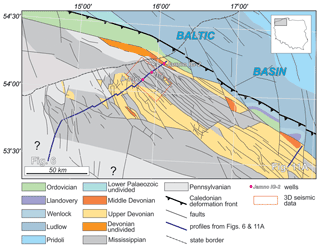
Figure 3Geological map of NW Poland without Permian, Mesozoic and Cenozoic (after Poprawa, 2019, supplemented).
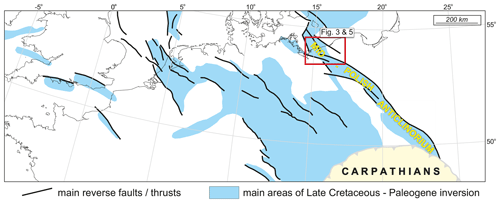
Figure 4Schematic extent of the Late Cretaceous–Paleogene foreland inversion in western and central Europe, compiled after Kley (2018), Krzywiec et al. (2021), Voigt et al. (2021), Ziegler (1990) and Ziegler et al. (2002).
The Caledonian orogenic belt in northern Poland ceased to exist due to Early Devonian uplift and widespread denudation (Dadlez, 1978; Poprawa, 2019). In the Variscan geological framework, the study area is located approximately 150 km NE from the front of the Variscan orogen that extends from the south-western UK across northern France, Belgium, northern Germany to Czechia, Poland and western Ukraine, and then on to Romania, Bulgaria and Turkey (Fig. 2; e.g. Martínez Catalan et al., 2020; Franke, 2014; Kröner et al., 2008; Krzywiec and Kufrasa, 2022; Laurent et al., 2021; Mazur et al., 2020; Okay and Topuz, 2017; Warr, 2012). The most external part of the Variscan orogen, i.e. the Rhenohercynian zone, forms a foreland fold-and-thrust belt built of the deformed Devonian–Carboniferous sedimentary succession deposited along the southern margin of Laurussia and subjected to progressive thrusting and folding (Oncken et al., 1999). The final emplacement of the Variscan fold-and-thrust belt onto its foreland plate took place in the late Carboniferous (Kröner et al., 2008; Mazur et al., 2010, 2020). It led to the regional flexure of the foreland plate and formation of extensive Carboniferous foreland basin filled with a thick synorogenic sedimentary succession (Burgess and Gayer, 2000; Deckers and Rombaut, 2021; Franke, 2014; Kombrink et al., 2010; Leveridge and Hartley, 2006; Maynard et al., 1997; McCann, 1999; McCann et al., 2008; Narkiewicz, 2007, 2020; Opluštil and Cleal, 2007; Tomek et al., 2019). Final stages of evolution of the external Variscan fold-and-thrust belt were associated with a widespread inversion of Palaeozoic basins located within its foreland (e.g. Corfield et al., 1996; Glen et al., 2005; Peace and Besly, 1997; Pharaoh et al., 2020; Shail and Leveridge, 2009; Smith, 1999; von Hartmann, 2003). Until recently, no Variscan inversion structures have been recognised within the NE part of the Variscan foreland, including NW Poland, mainly because of low quality of sub-salt seismic imaging of the sub-Zechstein interval. Relatively intense compressional deformations were documented in the area devoid of the Zechstein evaporitic cover in SE Poland and western Ukraine within the Lublin–Lviv Basin (Krzywiec et al., 2017a, b; Kufrasa et al., 2020; Tomaszczyk and Jarosiński, 2017; Zayats, 2015) and adjacent areas including the Holy Cross Mountains (e.g. Czarnocki, 1957; Lamarche et al., 1999; Konon, 2006, 2007). However, they have been recently interpreted as belonging to the frontal Variscan fold-and-thrust belt rather than being foreland inversion structures (see Krzywiec et al., 2017a, b; Kufrasa et al., 2020; Mazur et al., 2020, and references therein).
The upper Palaeozoic sedimentary cover within the study area consists of the (upper Emsian–?) Middle Devonian to lower Carboniferous (Mississippian) sediments deposited within the western Pomeranian Basin (Figs. 2, 3). This succession is composed of mainly clastic and carbonate sediments, with subordinate evaporites (Lipiec and Matyja, 1998; Narkiewicz, 2007, 2020; Narkiewicz et al., 1998; Matyja, 1993, 1998, 2006, 2008; Muszyński et al., 1996). Late Devonian–early Carboniferous formation of sedimentary cover of western Pomerania could be associated with regional extension and subsidence along the Laurussia southern margin (Smit et al., 2018).
The Pennsylvanian in the western Pomeranian Basin is developed only locally in the northern part of the basin (Żelichowski, 1995; Matyja, 2006; Kuberska et al., 2007). Also locally, uppermost Carboniferous to lowermost Permian volcanic rocks have been encountered by wells (Maliszewska et al., 2016).
The Variscan foreland basin ceased to exist in the latest Carboniferous due to regional post-orogenic uplift and erosion (Edel et al., 2018; McCann et al., 2006; Ziegler, 1990). This was followed by renewed subsidence and sedimentation related to formation of an extensive Permo-Mesozoic basin that covered the large part of Europe (Doornenbal and Stevenson, 2010; Littke et al., 2008; Pharaoh et al., 2010; Scheck-Wenderoth et al., 2008; van Wees et al., 2000; Ziegler, 1990). The Polish Basin, together with its axial most subsiding part, the Mid-Polish Trough, formed the easternmost segment of this vast sedimentary basin (Dadlez et al., 1995; Stephenson et al., 2003; Ziegler, 1990). The Polish Basin underwent long-term Mesozoic thermal subsidence, punctuated by three major pulses of extension-related accelerated tectonic subsidence: during late Permian to Early Triassic times, in the Late Jurassic (Oxfordian to Kimmeridgian) and in the early Cenomanian (Dadlez et al., 1995; Stephenson et al., 2003). Evolution of the NW and central segments of the Polish Basin, where a thick Zechstein evaporitic cover developed, was characterised by regional mechanical decoupling between the sub-Zechstein Rotliegend and older substratum and the Mesozoic supra-Zechstein cover (cf. Krzywiec, 2006a, b; Krzywiec et al., 2019, 2021, 2006). This led to formation of a system of peripheral extensional structures located above the basin margins', including grabens or half-grabens detached in evaporites, and salt structures (Krzywiec, 2002a, 2012; Rowan and Krzywiec, 2014; Warsitzka et al., 2021).
Deposition in the Polish Basin started with the Rotliegend (Wordian–Wuchiapingian) continental clastic sediments that, however, are not present in the study area as it was located within the marginal part of the basin (Kiersnowski, 1998; Kiersnowski and Buniak, 2006; Krzywiec et al., 2017c). The Permian sedimentary cover of the area analysed in this paper is restricted to Zechstein (Wuchiapingian to Changshingian) evaporites (Wagner, 1994, 1998; Krzywiec et al., 2017c). They are overlain by a Triassic to Cretaceous clastic–carbonate sedimentary succession (cf. Dadlez and Marek, 1997; Krzywiec, 2006a; Ziegler, 1990).
The Polish Basin was inverted in the Late Cretaceous–Paleogene (Dadlez et al., 1995, 1997; Głuszyński and Aleksandrowski, 2021; Krzywiec, 2002b, 2006b; Krzywiec et al., 2009; Resak et al., 2008). This was associated with widespread inversion of the European foreland triggered by the Alpine–Carpathian collision and Iberian–European convergence (cf. Kley, 2018; Kley and Voigt, 2008; Kockel, 2003; Kossow and Krawczyk, 2002; Voigt et al., 2021; Mazur et al., 2005). Inversion was associated with substantial uplift and erosion of the axial part of the Polish Basin, i.e. the Mid-Polish Trough, which presently forms a regional anticlinal structure referred to as the Mid-Polish Anticlinorium (Swell), outlined by the Cenozoic subcrop of the Lower Cretaceous and older rocks (Fig. 4). Inversion commenced in the late Turonian and lasted until the Maastrichtian–Paleocene (e.g. Krzywiec, 2002a, b, 2006b; Krzywiec et al., 2018; Resak et al., 2008). Due to regional inversion-driven uplift of the Mid-Polish Anticlinorium, the Upper Cretaceous mostly syn-inversion succession is presently preserved only along its flanks. Increased Late Cretaceous subsidence, related to flexural bending of both flanks of the uplifted Mid-Polish Anticlinorium (cf. Hindle and Kley, 2021) and combined with globally high Cretaceous sea level, created relatively large accommodation space filled by syn-kinematic Upper Cretaceous strata. On the other hand, progressive growth of particular inversion structures, including also compressionally reactivated salt diapirs, led to localised reduction of accommodation space and erosion, associated with formation of growth strata characterised by thickness reductions, progressive unconformities and facies changes (Leszczyński, 2012, 2002; Krzywiec, 2002a, 2006b, 2012; Krzywiec and Stachowska, 2016; Krzywiec et al., 2009, 2018). Inversion-related uplift of the Mid-Polish Anticlinorium was associated with compressional reactivation of peripheral thin-skinned structures formed above the basin's flanks (e.g. Burliga et al., 2012; Krzywiec, 2002a, b). The Koszalin–Chojnice Zone (structure), located within the NE flank of the NW segment of the Mid-Polish Anticlinorium (Figs. 5, 6) and analysed in this paper (see below), is one of these peripheral structures that underwent substantial inversion well documented by seismic data (cf. Krzywiec, 2006a, b).
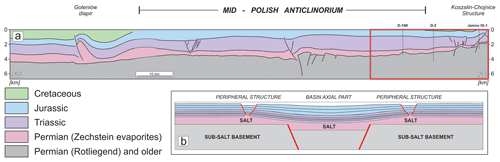
Figure 6(a) Regional seismo-geological transect illustrating structure of the Mid-Polish Anticlinorium (swell) in NW Poland (cf. Krzywiec, 2006a; Krzywiec et al., 2006). Red rectangle: part of the regional transect that was used to construct balanced model shown in Fig. 8. (b) Schematic model of a decoupled sedimentary basin developed above a thick salt layer during thick-skinned sub-salt extension (modified after Withjack and Callaway, 2000; see also Krzywiec, 2006b, 2012). Peripheral structures developed within the supra-salt sedimentary cover during decoupled extension often focus thin-skinned compressional deformation during ensuing basin inversion (cf. Burliga et al., 2012; Krzywiec, 2002a, 2012).
The Cenozoic unconsolidated, mostly clastic sediments of small thickness, generally not exceeding 200 m, unconformably cover the inverted and eroded Polish Basin (Piwocki and Kramarska, 2004; Jarosiński et al., 2009).
3.1 Seismic and well data
The seismic data used in this study included legacy 2-D data and newly acquired 3-D data. The regional seismo-geological transect shown in Fig. 6 was assembled from several 2-D profiles and then depth converted using velocity data from deep wells. The studied NE segment of this transect imaged the NE edge of the Mid-Polish Anticlinorium and the Koszalin–Chojnice structure – a peripheral structure developed within the NE flank of the Mid-Polish Trough/Anticlinorium. The 2-D seismic data in this part of the basin, characterised by a relatively thin Zechstein cover, did image some structures within the sub-Zechstein succession, although relatively poor quality of seismic imaging did not allow constructing a reliable geological model for the deeper substratum (Fig. 7a; cf. Antonowicz et al., 1994). A major breakthrough was related to acquisition of high-quality 3-D seismic data that provided a clear sub-Zechstein image in the study area (Trela et al., 2011; Fig. 7b). The seismic data were available in the depth domain and calibrated by several wells that, however, drilled the entire Permo-Mesozoic cover but encountered only the topmost part of the sub-Permian (i.e. sub-Zechstein) substratum, providing rather limited stratigraphic information on deeper seismic horizons within the Drzewiany Graben, excellently imaged by the new 3-D seismic data. The D-2 well and some indirect evidence were used to estimate the age of the sedimentary infill of this graben as Upper Devonian (Frasnian–Famennian?) to lower Carboniferous (Tournaisian; see also below).
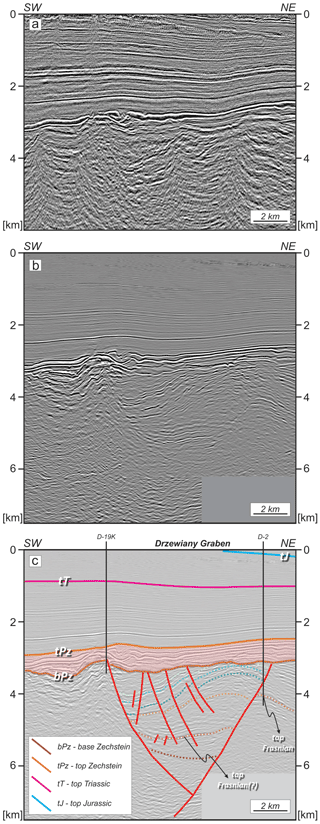
Figure 7Comparison of recently reprocessed vintage 1977 2-D seismic data (a) and recently acquired 3-D seismic data (b). Significant improvement in sub-salt (i.e. sub-Zechstein) seismic imaging is clearly visible. (c) The inverted Drzewiany Graben. Dotted brown lines: upper Devonian (Frasnian–Famennian?) horizons; dotted blue horizons: lower Carboniferous (Tournaisian) horizons calibrated by the D-2 well. Devonian stratigraphy within the Drzewiany Graben is conjectural only and based on similarity to the seismic characteristics of the Upper Devonian strata drilled by the D-2 well within the footwall of the Drzewiany master fault.
3.2 Structural restoration
Structural restoration was carried out along the cross-section that was located perpendicular to the strike of main structures imaged by the seismic data (Fig. 6). It was constructed using the NE part of the regional seismo-geological transect and two inlines extracted from the 3-D survey that was located in the central part of the cross-section.
Standard kinematic algorithms such as fault-bend folding and simple shear were used in order to obtain the pre-deformational geometry of key seismic horizons. Within the Drzewiany Graben, a shear angle was set to 50∘ and corresponds to the plane of maximum shear oriented parallel to minor normal fault surfaces (Dula, 1991; Xiao and Suppe, 1992). The relative timing of faults' activity was constrained based either on the presence of growth strata or cross-cutting relationships.
A maximum thickness of the syn-kinematic Upper Devonian–lower Carboniferous sedimentary sequence is deduced from the Drzewiany Graben, where it attains up to 4 km. Given that the most complete stratigraphic profile of the syn-kinematic strata is preserved within the SW part of the graben, it was used to approximate the currently missing portion of the Devonian–Carboniferous sedimentary rocks within the graben. Since little is known about a pre-deformation extent, geometry and thickness of the syn-tectonic strata at graben flanks, deposition of 50 m thick horizontal strata was assumed at each pre-inversion restoration step. It should be stressed though that, due to scarcity of data and widespread post-inversion erosion, reconstruction of the Devonian–Carboniferous cover outside the Drzewiany Graben was not the aim of this balancing exercise. The cross-sectional shape of the unconformity at the base of Permian was approximated by a regional trend line that connects the local depressions along this horizon. Flattening of the reference line resulted in preserving morphology of the unconformity. The initial thickness of Cretaceous sedimentary sequence eroded after the Alpine inversion was reconstructed using published palaeothickness maps (Leszczyński, 2002, 2012).
Modifications and improvements of the initial seismic interpretation were iteratively introduced until a satisfactory fit of the kinematic model to seismic and well data was achieved. The quality of the restored cross-section was verified via forward modelling by successively adding strain to the restored bed geometry, until the present-day shape of the horizons was obtained.
4.1 Seismic and well data
Well D-2 calibrated the Permian succession and provided partial stratigraphic information about the upper Palaeozoic infill of the Drzewiany Graben (Fig. 7c). It drilled Tournaisian strata within the hangingwall of the master fault of the graben, went through the fault, and encountered Frasnian–Famennian within the footwall. Upper Devonian was interpreted within the axial part of the graben using similarity of seismic horizons from the footwall and the hangingwall. Thickening of the large part of the sedimentary infill of the Drzewiany Graben towards the master fault suggests its syn-depositional activity during extension, similarly to the model shown in Fig. 1. On the other hand, the present-day geometry of the infill, in particular an anticline above the master fault, suggests substantial inversion (cf. Fig. 1).
The 2-D seismic data provided information on the regional present-day geometry of the Permian succession along the NE edge of the Mid-Polish Anticlinorium (Fig. 6). The Koszalin–Chojnice structure was interpreted as a thin-skinned anticlinal structure developed above the listric reverse fault rooted within the Zechstein evaporites, which is compatible with an overall geometry of this structure imaged by a large number of good-quality seismic data (cf. Krzywiec, 2006b, 2012; see also below).
4.2 Cross-section balancing
Cross-section restoration permitted us to distinguish four major deformation events in the tectonic evolution of the Drzewiany Graben (Fig. 8). According to the most plausible scenario, the Drzewiany Graben formed in response to Late Devonian–early Carboniferous NE–SW-oriented horizontal extension (Fig. 8b–i). Tectonic activity of the two conjugate, oppositely dipping graben-bounding normal faults created accommodation space successively filled with syn-tectonic deposits. A heterogeneous displacement along the master faults resulted in vertical variation in the geometry of growth strata within the graben: antithetic rotation and thickening toward the northeast is the most pronounced at the basal section of the syn-kinematic sedimentary sequence, as opposed to the youngest subparallel layers maintaining almost constant thicknesses (Fig. 8g–h). During the final stage of the extensional evolution of the Drzewiany Graben, the syn-tectonic strata were disrupted by secondary normal faults (Fig. 8i; cf. Jagger and McClay, 2018; McClay, 1995; McClay and Scott, 1991). The estimated total amount of the Late Devonian–early Carboniferous horizontal extension responsible for formation of the Drzewiany Graben was as high as 10 km (Fig. 8). However, this should be regarded as a minimum value as thickness of the post-Tournaisian strata that could have been deposited in this area is not known. In addition, the assumed thickness of the Tournaisian is a minimum estimate.
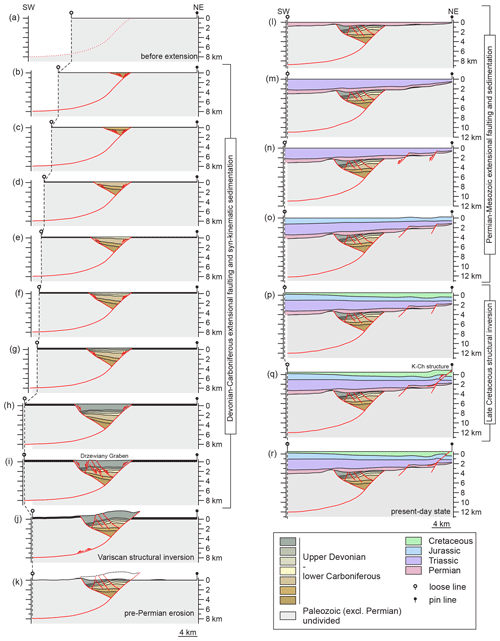
Figure 8Balanced model constructed for the NE part of the regional transect from Fig. 6a. It illustrates Late Devonian (Frasnian–Famennian?)–early Carboniferous (Tournaisian) extension and subsidence (a–i), late Carboniferous inversion followed by post-inversion erosion (j–k), Permo-Mesozoic subsidence within the peripheral part of the Mid-Polish Trough (l–o), Late Cretaceous–Paleogene inversion and uplift (p–q), and present-day geometry (r). K-Ch structure: the Koszalin–Chojnice structure.
The following phase of the Variscan structural inversion affected only the SW-dipping master fault by inducing 2 km of reverse displacement (Fig. 8j). The remaining normal faults do not show any seismic-scale signs of compressional reactivation. The syn-extensional sedimentary infill of the Drzewiany Graben, occupying a proximal portion of the hanging wall was then folded due to material translation over the underlying concave master fault. As a result, an asymmetric, open anticline with approximately 1.3 km of structural relief was produced (Fig. 8j). Its topmost part, together with growth strata that might have been deposited during inversion, was removed due to syn- to post-inversion erosion.
The subsidence centre of the Polish Basin, illustrated in Fig. 8l–o, developed SW from the analysed profile, within the Mid-Polish Trough, i.e. in the area where currently the Mid-Polish Anticlinorium, formed by the second inversion event, is located (cf. Fig. 6). Subsidence within the Mid-Polish Trough led to tilt of the pre-Permian strata by approximately 4∘ to the SW. It generated accommodation space that was successively filled in with sedimentary rocks thickening to the SW (i.e. towards the basin depocentre). Normal faulting related to the basin formation only slightly affected the study area by growth of new SW-dipping faults beneath the Zechstein evaporitic cover. It should be noted that faults outlining the inverted Drzewiany Graben were not reactivated during this tectonic phase. This deformation event yielded up to 500 m of horizontal stretching.
Evolution of the Permian–Mesozoic basin was terminated by the Late Cretaceous–Paleocene inversion that is the youngest major deformation stage discernible in the study area. It was caused by the NE–SW-oriented compression and formation of the thin-skinned anticlinal Koszalin–Chojnice structure (Fig. 8q; cf. Krzywiec, 2006a; Krzywiec et al., 2021). Its location might have been triggered by a buttressing effect of the sub-Zechstein basement steps related to small normal faults that were not reactivated during the inversion. Growth of the fault-related anticline was associated with approximately 500 m of horizontal shortening. Post-inversion erosion removed the topmost part of the Koszalin–Chojnice structure and part of the Upper Cretaceous syn-inversion cover. The Cenozoic post-inversion strata in this area are of negligible thickness and are not considered in this model.
The quantitative, balanced model that was prepared using seismic and well data from NW Poland depicted four main tectonic phases: (1) Late Devonian–early Carboniferous extension, (2) late Carboniferous inversion, (3) Permian subsidence and (4) Late Cretaceous–Paleogene inversion. Decoupling between two inversion events was caused by Zechstein evaporites. All these four phases will be discussed below in a regional context of geological evolution. A regional conceptual model depicting pre-Permian Palaeozoic evolution of the study area is shown in Fig. 9. Its eastern part is based on regional seismic profile Pl-5400 of the PolandSPAN® survey acquired above the Caledonian foredeep, i.e. the Baltic Basin (Krzywiec et al., 2014; Mazur et al., 2016); its western part is based on seismic data presented in this paper.
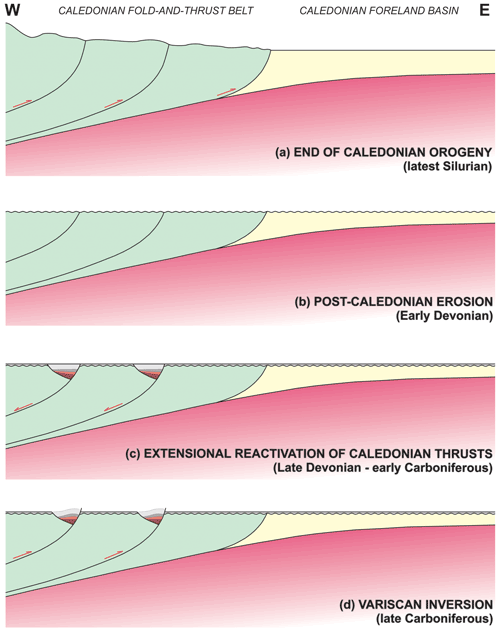
Figure 9Regional conceptual model showing four main stages of Palaeozoic tectono-stratigraphic evolution of northern Poland.
Two questions could be formulated regarding phase (1), i.e. the Late Devonian–early Carboniferous extension: (a) why was it focused in this area, and (b) what was the causal regional mechanism that led to this event? As it was described above, the western Pomeranian Basin developed above the frontal part of the thin-skinned Caledonian orogenic belt (Krzywiec et al., 2014; Mazur et al., 2016; see also Dadlez, 1978; Katzung et al., 1993; Podhalańska and Modliński, 2006; Poprawa, 2006; Znosko, 1965; Żaba and Poprawa, 2006). Reactivation of thrust structures as normal faults has been well documented in various orogenic belts (e.g. Corti et al., 2006; Deng et al., 2021; Faccenna et al., 1995; Fazlikhani et al., 2022; Tari et al., 2021; Withjack et al., 2010). Often, such reactivation is associated with formation of half-grabens with syn-kinematic deposition focused above hangingwall of such asymmetric extensional system, and formation of growth strata characterised by divergent stratal pattern and thickening towards the master fault, as shown in the middle panels of Fig. 1a, b and c (cf. Babaahmadi et al., 2018; Constenius, 1982, 1996; Powell and Williams, 1989; Tari et al., 2021; Velasco et al., 2010). As described by, e.g. Ivins et al. (1990), dips of many normal faults shallowing with depth are caused by the reactivation of pre-existing thrust faults of underlying thrust belts. All these features are compatible with characteristics of extensional Late Devonian–early Carboniferous half-grabens illustrated in Fig. 8b–i, including both their syn-kinematic sedimentary infill as well as geometry of the master fault. This fault could have been inherited from the Caledonian thrust belt although unequivocal seismic evidence of that is currently lacking. Late Palaeozoic extensional grabens have been widely documented in different parts of the extensionally reactivated Caledonides (e.g. Coward et al., 1987; Fossen, 2010; Koehl et al., 2018; Norton et al., 1987; Osmundsen and Andersen, 2001; Rowan and Jarvie, 2020; Scisciani et al., 2021; Séranne et al., 1989; Stemmerik, 2000). Such grabens, very similar to the Drzewiany Graben, located above the Caledonian thin-skinned fold-and-thrust belt, have been also documented using deep seismic data in the south-western Baltic Sea, NW from the western Pomeranian Basin (Lassen et al., 2001). In NW Poland, extensional reactivation of low-angle thrusts of the Caledonian thin-skinned orogenic wedge, characterised by rather large thickness (cf. Krzywiec et al., 2014; Mazur et al., 2016), explains both a listric geometry of the master fault that governed the evolution of the Drzewiany Graben as well as its thin-skinned character (Fig. 9; see also Fig. 1b). Late Devonian–early Carboniferous extension that reactivated Caledonian orogenic wedge in NW Poland coincided with a regional extensional phase that affected the southern margin of Laurussia (cf. Smit et al., 2018).
Inversion tectonics and reactivation of basement faults and fracture zones within the forelands of orogenic belts is a well-known process (cf. Ziegler et al., 2002). Well-documented examples include the Apennines (Costa et al., 2021; Scisciani, 2009), the Andes (Delgado et al., 2012; Bilmes et al., 2013; Iaffa et al., 2011) and the Alps (Schori et al., 2021). Deformation within the Variscan orogenic belt also have expressions within the Variscan foreland – a whole array of inverted faults and basins in front of the Variscan belt have been documented in the southern UK (Chadwick and Evans, 2005; Corfield et al., 1996; Glen et al., 2005; Peace and Besly, 1997; Shail and Leveridge, 2009; Smith, 1999). Figure 10 shows an interpreted seismic profile across the Eakring anticline that documents substantial Dinantian (Tournaisian–Visean) extension and subsidence, followed by late Carboniferous Variscan inversion, post-inversion erosion and Permo-Triassic sedimentation. In this case, there was no Late Devonian extension although the lack of deep wells and inferior seismic imaging at deeper level probably does not preclude this. The Eakring anticline could be directly compared to the inverted Drzewiany Graben, both in terms of an overall geometry and main stages of development of Variscan inversion structures. There are also similar sub-Zechstein Variscan structures imaged on seismic data in northern Germany (von Hartmann, 2003).
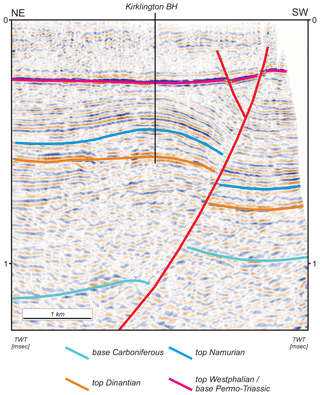
Figure 10Interpreted seismic profile across the Eakring anticline located approximately 150 km towards the north from the Variscan orogenic front in the southern UK (based on Chadwick and Evans, 2005).
Late Cretaceous inversion in the vicinity of NW Poland has been documented by numerous authors (Deeks and Thomas, 1995; Krzywiec et al., 2003, 2021; Mazur et al., 2005; Meissner et al., 2002; Sopher et al., 2016; Seidel et al., 2018; Deutschmann et al., 2018). Seismic examples from areas without Zechstein evaporites show deeply rooted reverse faults, along which basement blocks have been uplifted. However, different geometries are observed in the areas where Zechstein evaporites were deposited. The evaporites led to regional mechanical decoupling between the sub-evaporitic basement and supra-evaporitic cover, both during extension as well as inversion (e.g. Ahlrichs et al., 2020; Betz et al., 1987; Burliga et al., 2012; Dooley and Hudec, 2020; Lohr et al., 2007; Marsh et al., 2010; Soto et al., 2007; Stewart, 1999; Withjack and Callaway, 2000). In our study area, a thin-skinned inversion structure – the Koszalin–Chojnice structure – has been documented by seismic data. Due to deep post-inversion erosion, the top of this inversion anticline and associated inversion-related growth strata were removed. Better examples of the same structure are provided by seismic data from its more south-eastern segment, where relatively thick Upper Cretaceous succession is still preserved (Fig. 11a; cf. also Krzywiec, 2006b, 2012). Similar structures have been documented in the southern UK (e.g. Chadwick and Evans, 2005; Cosgrove et al., 2021). One of them is the Weymouth anticline, shown in Fig. 11b. It evolved from Early Jurassic to Early Cretaceous due to detached thin-skinned listric normal faulting above the morphologically varied sub-salt basement. For this structure, Cenozoic (Miocene) inversion was postulated (Chadwick and Evans, 2005).
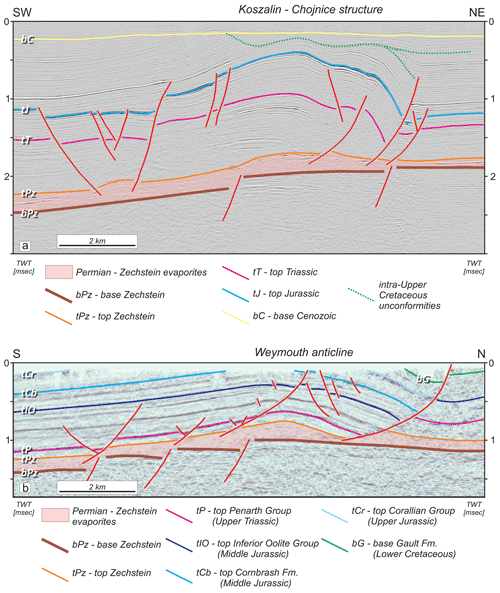
Figure 11Thin-skinned inversion structures detached within the Zechstein evaporites: the Koszalin–Chojnice structure from NW Poland (a; cf. Krzywiec, 2006b, 2012) and the Weymouth anticline from the southern UK (b; based on Chadwick and Evans, 2005, partly reinterpreted). Both seismic lines are displayed with the same horizontal and vertical scale so certain structural features could be directly compared.
As it was described and illustrated above, the area analysed in this paper underwent two phases of inversion. Repeated extension and compression occurring along the same faults have been described by many authors (e.g. Bosworth and Tari, 2021; Dichiarante et al., 2020; Minguely et al., 2010). In our case, the presence of Zechstein evaporites resulted in mechanical decoupling between the Devonian–Carboniferous and Triassic–Cretaceous levels, and, despite their proximity to one another, both inversion structures evolved independently. Variscan inversion was associated with compressional reactivation of a listric normal fault that might have originally originated as a Caledonian thrust. Alpine (Late Cretaceous–Paleocene) inversion was associated with listric thin-skinned reverse faulting detached within the Zechstein evaporites. Both inversion structures are compatible with certain elements of the classic inversion model by Bally (1984) but collectively form a complex, superimposed multilayer decoupled inversion system.
Seismic and well data (excluding Jamno IG-2 well) used in this study are confidential and not available publicly. Data from Jamno IG-2 well could be accessed via the Central Geological Database and National Geological Archive maintained by the Polish Geological Institute (http://geoportal.pgi.gov.pl, Polish Geological Institute, 2022).
PK wrote most of the text, prepared most of the figures and compiled the paper. MK prepared the balanced model and the relevant part of the text and Fig. 8. PP and SM provided expertise on regional geology. MK and PŚ were instrumental in providing the data used in this study and were involved in discussions on interpretation of seismic data.
The contact author has declared that neither they nor their co-authors have any competing interests.
Publisher’s note: Copernicus Publications remains neutral with regard to jurisdictional claims in published maps and institutional affiliations.
This article is part of the special issue “Inversion tectonics – 30 years later”. It is a result of the EGU General Assembly 2019, Vienna, Austria, 7–12 April 2019.
PGNiG S.A. kindly provided all the seismic and well data used in this study. Seismic data were acquired and processed by Geofizyka Toruń S.A. IHS Markit and Petroleum Experts are acknowledged for providing software for seismic data interpretation and cross-section balancing, respectively. Piotr Krzywiec would like to thank colleagues from Geofizyka Toruń S.A., especially Paweł Pomianowski and Mariusz Łukaszewski, for stimulating discussions regarding various aspects of acquisition and processing of seismic data from the Pomeranian region. Gabor Tari and Mark Cooper are thanked for their reviews that helped to finally shape this paper.
This study was supported by PGNiG S.A.; regional analysis was completed within NCN grant no. UMO-2015/17/B/ST10/03411.
This paper was edited by Jonas Kley and reviewed by Gabor Tari and Mark Cooper.
Ahlrichs, N., Hübscher, C., Noack, V., Schnabel, M., Damm, V., and Krawczyk, C. M.: Structural Evolution at the Northeast North German Basin Margin: From Initial Triassic Salt Movement to Late Cretaceous-Cenozoic Remobilization, Tectonics, 39, e2019TC005927, https://doi.org/10.1029/2019TC005927, 2020.
Antonowicz, L., Iwanowska, E., and Rendak, A.: Tensional tectonics in the Pomeranian section of the T-T Zone and the implications for hydrocarbon exploration, Kwart. Geol., 38, 289–305, 1994.
Babaahmadi, A., Sliwa, R., Esterle, J., and Rosenbaum, G.: The evolution of a Late Cretaceous–Cenozoic intraplate basin (Duaringa Basin), eastern Australia: evidence for the negative inversion of a pre-existing fold–thrust belt, Int. J. Earth Sci., 107, 1895–1910, https://doi.org/10.1007/s00531-017-1577-3, 2018.
Bally, A. W.: Tectogenese et sismique reflexion, B. Soc. Geol. Fr., S7-XXVI, 279–285, https://doi.org/10.2113/gssgfbull.s7-xxvi.2.279, 1984 (in French with English summary).
Betz, D., Führer, F., Greiner, G., and Plein, E.: Evolution of the Lower Saxony Basin, Tectonophysics, 137, 127–170, https://doi.org/10.1016/0040-1951(87)90319-2, 1987.
Bilmes, A., D'Elia, L., Franzese, J. R., Veiga, G. D., and Hernández, M.: Miocene block uplift and basin formation in the Patagonian foreland: The Gastre Basin, Argentina, Tectonophysics, 601, 98–111, https://doi.org/10.1016/j.tecto.2013.05.001, 2013.
Bonini, M., Sani, F., and Antonielli, B.: Basin inversion and contractional reactivation of inherited normal faults: A review based on previous and new experimental models, Tectonophysics, 522–523, 55–88, https://doi.org/10.1016/j.tecto.2011.11.014, 2012.
Bosworth, W. and Tari, G.: Hydrocarbon accumulation in basins with multiple phases of extension and inversion: examples from the Western Desert (Egypt) and the western Black Sea, Solid Earth, 12, 59–77, https://doi.org/10.5194/se-12-59-2021, 2021.
Brun, J.-P. and Nalpas, T.: Graben inversion in nature and experiments, Tectonics, 15, 677–687, https://doi.org/10.1029/95TC03853, 1996.
Buchanan, J. G. and Buchanan, P. G. (Eds.): Basin Inversion, Geol. Soc. Spec. Publ., 88, Geological Society, London, UK, 596 pp., ISBN-13 978-1-8977-9929-1, 1995.
Buiter, S. J. H. and Pfiffner, A. O.: Numerical models of the inversion of half-graben basins, Tectonics, 22, 1057, https://doi.org/10.1029/2002TC001417, 2003.
Buiter, S. J. H., Pfiffner, O. A., and Beaumont, C.: Inversion of extensional sedimentary basins: A numerical evaluation of the localization of shortening, Earth Planet. Sc. Lett., 288, 492–504, https://doi.org/10.1016/j.epsl.2009.10.011, 2009.
Burgess, P. M. and Gayer, R. A.: Late Carboniferous tectonic subsidence in South Wales: Implications for Variscan basin evolution and tectonic history in SW Britain, J. Geol. Soc. Lond., 157, 93–104, https://doi.org/10.1144/jgs.157.1.93, 2000.
Burliga, S., Koyi, H. A., and Krzywiec, P.: Modelling cover deformation and decoupling during inversion, using the Mid-Polish Trough as a case study, J. Struct. Geol., 42, 62–73, https://doi.org/10.1016/j.jsg.2012.06.013, 2012.
Chadwick, R. A. and Evans, D. J.: A seismic atlas of southern Britain – Images of subsurface structure, British Geological Survey Occasional Publication, 7, British Geological Survey, Keyworth., 196 pp., ISBN 0852725124, 2005.
Chadwick, R. A. and Smith, N. J. P.: Evidence of negative structural inversion beneath central England from new seismic reflection data, J. Geol. Soc. Lond., 145, 519–522, 1988.
Cloetingh, S., Beekman, F., Ziegler, P. A., van Wees, J.-D., and Sokoutis, D.: Post-rift compressional reactivation potential of passive margins and extensional basins, Geol. Soc. Lond. Spec. Publ., 306, 27–70, https://doi.org/10.1144/SP306.2, 2008.
Connors, D. C. and Houseknecht, D. W.: Structural Inheritance in the Chukchi Shelf, Alaska, Mar. Petrol. Geol., in press, 2022.
Constenius, K. N.: Relationship between the Kishenehn basin, and the Flathead listric normal fault system and Lewis thrust salient, Geologic Studies of the Cordilleran Thrust Belt, Rocky Mountain Association of Geologists, 2, 817–830, 1982.
Constenius, K. N.: Late Paleogene extensional collapse of the Cordilleran foreland fold and thrust belt, Geol. Soc. Am. Bull., 108, 20–39, https://doi.org/10.1130/0016-7606(1996)108<0020:LPECOT>2.3.CO;2, 1996.
Cooper, M. and Warren, M. J.: The geometric characteristics, genesis and petroleum significance of inversion structures, Geol. Soc. Lond. Spec. Publ., 335, 827–846, https://doi.org/10.1144/SP335.33, 2010.
Cooper, M. and Warren, M. J.: Inverted fault systems and inversion tectonic settings, in: Regional Geology and Tectonics: Principles of Geologic Analysis, edited by: Scarselli, N., Adam, J., Chiarella, D., Roberts, D. G., and Bally, A. W., Elsevier, Amsterdam, the Netherlands, 169–204, https://doi.org/10.1016/B978-0-444-64134-2.00009-2, 2020.
Cooper, M. A. and Williams, G. D.: Inversion tectonics, Geol. Soc. Lond. Spec. Publ., 44, 375 pp., 1989.
Cooper, M. A., Williams, G. D., de Graciansky, P. C., Murphy, R. W., Needham, T., de Paor, D., Stoneley, R., Todd, S. P., Turner, J. P., and Ziegler, P. A.: Inversion tectonics – a discussion, Geol. Soc. Lond. Spec. Publ., 44, 335–347, https://doi.org/10.1144/GSL.SP.1989.044.01.18, 1989.
Corfield, S. M., Gawthorpe, R. L., Gage, M., Fraser, A. J., and Besly, B. M.: Inversion tectonics of the Variscan foreland of the British Isles, J. Geol. Soc. Lond., 153, 17–32, https://doi.org/10.1144/gsjgs.153.1.0017, 1996.
Corti, G., Lucia, S., Bonini, M., Sani, F., and Mazzarini, F.: Interaction between normal faults and pre-existing thrust systems in analogue models, Geol. Soc. Lond. Spec. Publ., 253, 65–78, https://doi.org/10.1144/GSL.SP.2006.253.01.03, 2006.
Cosgrove, J. W., Morgan, T. O., and Ghail, R.: The deformation history of southern England, and its implications for ground engineering in the London Basin, Q. J. Eng. Geol. Hydroge., 55, qjegh2020-144, https://doi.org/10.1144/qjegh2020-144, 2021.
Costa, M., Chicco, J., Invernizzi, C., Teloni, S., and Pierantoni, P. P.: Plio–Quaternary Structural Evolution of the Outer Sector of the Marche Apennines South of the Conero Promontory, Italy, Geosciences, 11, 184, https://doi.org/10.3390/geosciences11050184, 2021.
Coward, M. P., Enfield, M. A., and Fischer, M. W.: Devonian basins of Northern Scotland: extension and inversion related to Late Caledonian – Variscan tectonics, Geol. Soc. Lond. Spec. Publ., 44, 275–308, https://doi.org/10.1144/GSL.SP.1989.044.01.16, 1987.
Czarnocki, J.: Tectonics of the Święty Krzyż Mountains, Stratigraphy and tectonics of the Święty Krzyż Mountains, Pr. Geol. Inst. Geol., 18, 11–133, 1957 (in Polish with English summary).
Dadlez, R.: Sub-Permian rock complexes in the Koszalin–Chojnice zone, Geol. Q., 22, 269–301, 1978 (in Polish with English summary).
Dadlez, R. and Marek, S.: Development of the Permian and Mesozoic basins, Pr. Inst. Geol., 153, 403–409, 1997 (in Polish with English summary).
Dadlez, R., Narkiewicz, M., Stephenson, R. A., Visser, M. T. M., and van Wees, J. D.: Tectonic evolution of the Mid-Polish Trough: Modelling implications and significance for central European geology, Tectonophysics, 252, 179–195, https://doi.org/10.1016/0040-1951(95)00104-2, 1995.
Dadlez, R., Jóźwiak, W., and Młynarski, S.: Subsidence and inversion in the western part of Polish Basin – data from seismic velocities, Kwart. Geol., 41, 197–208, 1997.
Dadlez, R., Marek, S., and Pokorski, J.: Geological map of Poland without Cainozoic deposits, 1 : 1 000 000, Państwowy Instytut Geologiczny, Warszawa, Poland, 2000.
Deckers, J. and Rombaut, B.: Late Carboniferous differential subsidence in the Campine Basin as part of the Variscan foreland system, Int. J. Earth Sci., 110, 875–888, https://doi.org/10.1007/s00531-021-01996-z, 2021.
Deeks, N. R. and Thomas, S. A.: Basin inversion in a strike-slip regime: The Tornquist Zone, Southern Baltic Sea, Geol. Soc. Lond. Spec. Publ., 88, 319–338, https://doi.org/10.1144/GSL.SP.1995.088.01.18, 1995.
Delgado, A., Mora, A., and Reyes-Harker, A.: Deformation partitioning in the Llanos foreland basin during the Cenozoic and its correlation with mountain building in the hinterland, J. S. Am. Earth Sci., 39, 228–244, https://doi.org/10.1016/j.jsames.2012.04.011, 2012.
Del Ventisette, C., Bonini, M., Maestrelli, D., Sani, F., Iavarone, E., and Montanari, D.: 3D-thrust fault pattern control on negative inversion: An analogue modelling perspective on central Italy, J. Struct. Geol., 143, 104254, https://doi.org/10.1016/j.jsg.2020.104254, 2021.
Deng, C., Zhu, R., Han, J., Shu, Y., Wu, Y., Hou, K., and Long, W.: Impact of basement thrust faults on low-angle normal faults and rift basin evolution: a case study in the Enping sag, Pearl River Basin, Solid Earth, 12, 2327–2350, https://doi.org/10.5194/se-12-2327-2021, 2021.
Deutschmann, A., Meschede, M., and Obst, K.: Fault system evolution in the Baltic Sea area west of Rügen, NE Germany, Geol. Soc. Lond. Spec. Publ., 469, 83–98, https://doi.org/10.1144/SP469.24, 2018.
Dewey, J. F.: Kinematics and dynamics of basin inversion, in: Inversion Tectonics, edited by: Cooper, M. A. and Williams, G. D., Geol. Soc. Lond. Spec. Publ., 44, 352, https://doi.org/10.1144/GSL.SP.1989.044.01.20, 1989.
Dichiarante, A. M., Holdsworth, R. E., Dempsey, E. D., McCaffrey, K. J. W., and Utley, T. A. G.: Outcrop-scale manifestations of reactivation during multiple superimposed rifting and basin inversion events: the Devonian Orcadian Basin, northern Scotland, J. Geol. Soc., 178, jgs2020-089, https://doi.org/10.1144/jgs2020-089, 2020.
Di Domenica, A., Petricca, P., Trippetta, F., Carminati, E., and Calamita, F.: Investigating fault reactivation during multiple tectonic inversions through mechanical and numerical modeling: An application to the Central-Northern Apennines of Italy, J. Struct. Geol., 67, 167–185, https://doi.org/10.1016/j.jsg.2014.07.018, 2014.
Dooley, T. P. and Hudec, M. R.: Extension and inversion of salt-bearing rift systems, Solid Earth, 11, 1187–1204, https://doi.org/10.5194/se-11-1187-2020, 2020.
Doornenbal, H. and Stevenson, A. (Eds.): Petroleum Geological Atlas of the Southern Permian Basin Area, EAGE Publications BV, Houten, the Netherlands, 342 pp., ISBN 978-90-73781-61-0, 2010.
Dula, W. F. J.: Geometric Models of Listric Normal Faults and Rollover Folds (1), Am. Assoc. Petr. Geol. B., 75, 1609–1625, https://doi.org/10.1306/0C9B29B1-1710-11D7-8645000102C1865D, 1991.
Edel, J. B., Schulmann, K., Lexa, O., and Lardeaux, J. M.: Late Palaeozoic palaeomagnetic and tectonic constraints for amalgamation of Pangea supercontinent in the European Variscan belt, Earth-Sci. Rev., 177, 589–612, https://doi.org/10.1016/j.earscirev.2017.12.007, 2018.
Faccenna, C., Nalpas, T., Brun, J.-P., Davy, P., and Bosi, V.: The influence of pre-existing thrust faults on normal fault geometry in nature and in experiments, J. Struct. Geol., 17, 1139–1149, https://doi.org/10.1016/0191-8141(95)00008-2, 1995.
Fazlikhani, H., Bauer, W., and Stollhofen, H.: Variscan structures and their control on latest to post-Variscan basin architecture: insights from the westernmost Bohemian Massif and southeastern Germany, Solid Earth, 13, 393–416, https://doi.org/10.5194/se-13-393-2022, 2022.
Fossen, H.: Extensional tectonics in the North Atlantic Caledonides: a regional view, Geol. Soc. Lond. Spec. Publ., 335, 767–793, https://doi.org/10.1144/SP335.31, 2010.
Franke, W.: Topography of the Variscan orogen in Europe: Failed–not collapsed, Int. J. Earth Sci., 103, 1471–1499, https://doi.org/10.1007/s00531-014-1014-9, 2014.
Fyhn, M. B. W., Cuong, T. D., Hoang, B. H., Hovikoski, J., Olivarius, M., Tuan, N. Q., Tung, N. T., Huyen, N. T., Cuong T. X., Nytoft, H. P., Abatzis, I., and Nielsen, L. H.: Linking Paleogene rifting and inversion in the northern Song Hong and Beibuwan Basins, Vietnam, with left-lateral motion on the Ailao Shan-Red River Shear Zone, Tectonics, 37, 2559–2585, https://doi.org/10.1029/2018TC005090, 2018.
Gibson, G. M. and Edwards, S.: Basin inversion and structural architecture as constraints on fluid flow and Pb–Zn mineralization in the Paleo–Mesoproterozoic sedimentary sequences of northern Australia, Solid Earth, 11, 1205–1226, https://doi.org/10.5194/se-11-1205-2020, 2020.
Glen, R. A., Hancock, P. L., and Whittaker, A.: Basin inversion by distributed deformation: The southern margin of the Bristol Channel Basin, England, J. Struct. Geol., 27, 2113–2134, https://doi.org/10.1016/j.jsg.2005.08.006, 2005.
Glennie, K. W. and Boegner, P. L. E.: Sole Pit inversion tectonics, in: Petroleum Geology of the Continental Shelf of Northwest Europe, edited by: Illing, L. V. and Hobson, G. D., Institute of Petroleum, London, 110–120, ISBN 0855-016566, 1981.
Głuszyński, A. and Aleksandrowski, P.: Late Cretaceous – Early Palaeogene inversion-related tectonic structures at the NE margin of the Bohemian Massif (SW Poland and northern Czechia), Solid Earth Discuss. [preprint], https://doi.org/10.5194/se-2021-99, in review, 2021.
Grad, M. and Polkowski, M.: Seismic basement in Poland, Int. J. Earth Sci., 105, 1199–1214, https://doi.org/10.1007/s00531-015-1233-8, 2016.
Grad, M., Guterch, A., and Mazur, S.: Seismic refraction evidence for crustal structure in the central part of the Trans-European Suture Zone in Poland, Geol. Soc. Lond. Spec. Publ., 201, 295–309, https://doi.org/10.1144/GSL.SP.2002.201.01.14, 2002.
Guterch, A. and Grad, M.: Lithospheric structure of the TESZ in Poland based on modern seismic experiments, Geol. Q., 50, 23–32, 2006.
Guterch, A., Grad, M., Thybo, H., and Keller, G. R.: POLONAISE '97 – an international seismic experiment between Precambrian and Variscan Europe in Poland, Tectonophysics, 314, 101–121, https://doi.org/10.1016/S0040-1951(99)00239-5, 1999.
Guterch, A., Wybraniec, S., Grad, M., Chadwick, R. A., Krawczyk, C. M., Ziegler, P. A., Thybo, H., and De Vos, W.: Crustal structure and structural framework, in: Petroleum Geological Atlas of the Southern Permian Basin Area, edited by: Doornenbal, H. and Stevenson, A., EAGE Publications BV, Houten, the Netherlands, 11–23, ISBN 978-90-73781-61-0, 2010.
Hansen, T. H., Clausen, O. R., and Andresen, K. J.: Thick- and thin-skinned basin inversion in the Danish Central Graben, North Sea – the role of deep evaporites and basement kinematics, Solid Earth, 12, 1719–1747, https://doi.org/10.5194/se-12-1719-2021, 2021.
Henk, A. and Nemčok, M.: Stress and fracture prediction in inverted half-graben structures, J. Struct. Geol., 30, 81–97, https://doi.org/10.1016/j.jsg.2007.10.006, 2008.
Hindle, D. and Kley, J.: The Subhercynian Basin: an example of an intraplate foreland basin due to a broken plate, Solid Earth, 12, 2425–2438, https://doi.org/10.5194/se-12-2425-2021, 2021.
Holdsworth, R. E., Butler, C. A., and Roberts, A. M.: The recognition of reactivation during continental deformation, J. Geol. Soc. Lond., 154, 73–78, https://doi.org/10.1144/gsjgs.154.1.0073, 1997.
Iaffa, D. N., Sàbat, F., Muñoz, J. A., Mon, R., and Gutierrez, A. A.: The role of inherited structures in a foreland basin evolution. The Metán Basin in NW Argentina, J. Struct. Geol., 33, 1816–1828, https://doi.org/10.1016/j.jsg.2011.09.005, 2011.
Ivins, E. R., Dixon, T. H., and Golombek, M. P.: Extensional reactivation of an abandoned thrust: a bound on shallowing in the brittle regime, J. Struct. Geol., 12, 303–314, https://doi.org/10.1016/0191-8141(90)90015-Q, 1990.
Jackson, C. A. L. and Larsen, E.: Temporal constraints on basin inversion provided by 3D seismic and well data: a case study from the South Viking Graben, offshore Norway, Basin Res., 20, 397–417, https://doi.org/10.1111/j.1365-2117.2008.00359.x, 2008.
Jackson, C.-L., Chua, S.-T., Bell, R., and Magee, C.: Structural style and early stage growth of inversion structures: 3D seismic insights from the Egersund Basin, offshore Norway, J. Struct. Geol., 46, 167–185, 2013.
Jagger, L. J. and McClay K. R.: Analogue modelling of inverted domino-style basement fault systems, Basin Res., 30, 363–381, https://doi.org/10.1111/bre.12224, 2018.
Jarosiński, M., Poprawa, P., and Ziegler, P. A.: Cenozoic dynamic evolution of the Polish Platform, Geol. Q., 53, 3–26, 2009.
Katzung, G., Giese, U., Walter, R., and Von Winterfeld, C.: The Rügen Caledonides, northeast Germany, Geol. Mag., 130, 725–730, https://doi.org/10.1017/S0016756800021038, 1993.
Kiersnowski, H.: Depositional architecture of the Rotliegend Basin in Poland, Pr. Państwowego Inst. Geol., 165, 113–128, 1998 (in Polish with English summary).
Kiersnowski, H. and Buniak, A.: Evolution of the Rotliegend Basin of northwestern Poland, Geol. Q., 50, 119–138, 2006.
Kley, J.: Timing and spatial patterns of Cretaceous and Cenozoic inversion in the Southern Permian Basin, Geol. Soc. Lond. Spec. Publ., 469, 19–31, https://doi.org/10.1144/SP469.12, 2018.
Kley, J. and Voigt, T.: Late Cretaceous intraplate thrusting in central Europe: Effect of Africa-Iberia-Europe convergence, not Alpine collision, Geology, 36, 839–842, https://doi.org/10.1130/G24930A.1, 2008.
Kockel, F.: Inversion structures in Central Europe – Expressions and reasons, an open discussion, Netherlands J. Geosci., 82, 351–366, https://doi.org/10.1017/S0016774600020187, 2003.
Koehl, J.-B. P., Bergh, S. G., Henningsen, T., and Faleide, J. I.: Middle to Late Devonian–Carboniferous collapse basins on the Finnmark Platform and in the southwesternmost Nordkapp basin, SW Barents Sea, Solid Earth, 9, 341–372, https://doi.org/10.5194/se-9-341-2018, 2018.
Kombrink, H., Besly, B. M., Collinson, J. D., Den Hartog Jager, D. G., Drozdzewski, G., Dusar, M., Hoth, P., Pagnier, H. J. M., Stemmerik, L., Waksmundzka, M. I., and Wrede, V.: Carboniferous, in: Petroleum Geological Atlas of the Southern Permian Basin Area, edited by: Doornenbal, H. and Stevenson, A., EAGE Publications BV, Houten, the Netherlands, 81–99, ISBN 978-90-73781-61-0, 2010.
Konon, A.: Buckle folding in the Kielce Unit, Holy Cross Mountains, central Poland, Acta Geol. Pol., 56, 375–405, 2006.
Konon, A.: Strike-slip faulting in the Kielce Unit, Holy Cross Mountains, central Poland, Acta Geol. Pol., 57, 415–441, 2007.
Kossow, D. and Krawczyk, C. M.: Structure and quantification of processes controlling the evolution of the inverted NE-German Basin, Mar. Petrol. Geol., 19, 601–618, https://doi.org/10.1016/S0264-8172(02)00032-6, 2002.
Krantz, R. W.: Normal fault geometry and fault reactivation in tectonic inversion experiments, Geol. Soc. Spec. Publ., 56, 219–229, 1991.
Kröner, U., Mansy, J.-L., Mazur, S., Aleksandrowski, P., Hann, H. P., Huckriede, H., Lacquement, F., Lamarche, J., Ledru, P., Pharaoh, T. C., Zedler, H., Zeh, A., and Zulauf, G.: Variscan tectonics, in: The Geology of Central Europe Volume 1: Precambrian and Palaeozoic, edited by: McCann, T., The Geological Society of London, London, UK, 599–664, https://doi.org/10.1144/CEV1P.11, 2008.
Krzywiec, P.: Oświno structure (NW Mid-Polish Trough) – salt diapir or inversion-related compressional structure?, Geol. Q., 46, 337–346, 2002a.
Krzywiec, P.: Mid-Polish Trough inversion – seismic examples, main mechanisms, and its relationship to the Alpine-Carpathian collision, Stephan Mueller Spec. Publ. Ser., 1, 151–165, https://doi.org/10.5194/smsps-1-151-2002, 2002b.
Krzywiec, P.: Triassic-Jurassic evolution of the Pomeranian segment of the Mid-Polish Trough – Basement tectonics and subsidence patterns, Geol. Q., 50, 139–150, 2006a.
Krzywiec, P.: Structural inversion of the Pomeranian and Kuiavian segments of the Mid-Polish Trough – Lateral variations in timing and structural style, Geol. Q., 50, 151–168, 2006b.
Krzywiec, P.: Mesozoic and Cenozoic evolution of salt structures within the Polish basin: An overview, Geol. Soc. Lond. Spec. Publ., 363, 381–394, https://doi.org/10.1144/SP363.17, 2012.
Krzywiec, P. and Kufrasa, M.: External Variscides in South-Eastern Poland and Western Ukraine, in: Geology of the Central European Variscides and its Avalonian-Cadomian precursors, edited by: Linnemann, U., Springer-Verlag, Berlin, Heidelberg, Germany, submitted, 2022.
Krzywiec, P. and Stachowska, A.: Late Cretaceous inversion of the NW segment of the Mid-Polish Trough – how marginal trough was formed, and does it matter at all?, Z. Dtsch. Ges. Geowiss., 167, 107–119, https://doi.org/10.1127/zdgg/2016/0068, 2016.
Krzywiec, P., Kramarska, R., and Zientara, P.: Strike-slip tectonics within the SW Baltic Sea and its relationship to the inversion of the Mid-Polish Trough – evidence from high-resolution seismic data, Tectonophysics, 373, 93–105, https://doi.org/10.1016/S0040-1951(03)00286-5, 2003.
Krzywiec, P., Wybraniec, S., and Petecki, Z.: Basement tectonics of the Mid-Polish trough in central and northern Poland – Results of analysis of seismic reflection, gravity and magnetic data, Pr. Państwowego Inst. Geol., 188, 107–130, 2006 (in Polish with English summary).
Krzywiec, P., Gutowski, J., Walaszczyk, I., Wróbel, G., and Wybraniec, S.: Tectonostratigraphic model of the Late Cretaceous inversion along the Nowe Miasto–Zawichost Fault Zone, SE Mid-Polish Trough, Geol. Q., 53, 27–48, 2009.
Krzywiec, P., Malinowski, M., Lis, P., Buffenmyer, V., and Lewandowski, M.: Lower Paleozoic basins developed above the East European Craton in Poland: new insight from regional high-effort seismic reflection data, SPE/EAGE European Unconventional Resources Conference and Exhibition, Vienna, extended abstract, SPE-167739-MS, 2014.
Krzywiec, P., Gągała, Mazur, S., Słonka, Ł., Kufrasa, M., Malinowski, M., Pietsch, K., and Golonka, J.: Variscan deformation along the Teisseyre-Tornquist Zone in SE Poland: Thick-skinned structural inheritance or thin-skinned thrusting?, Tectonophysics, 718, 83–91, https://doi.org/10.1016/j.tecto.2017.06.008, 2017a.
Krzywiec, P., Mazur, S., Gągała, Ł., Kufrasa, M., Lewandowski, M., Malinowski, M., and Buffenmyer, V.: Late Carboniferous thin-skinned compressional deformation above the SW edge of the East European Craton as revealed by reflection seismic and potential fields data – correlations with the Variscides and the Appalachians, in: Linkages and Feedbacks in Orogenic Processes, edited by: Law, R., Thigpen, R., Stowell, H., and Merschat, A., Geol. Soc. Am. Mem., 213, 353–372, https://doi.org/10.1130/2017.2013(14), 2017b.
Krzywiec, P., Peryt, T. M., Kiersnowski, H., Pomianowski, P., Czapowski, G., and Kwolek, K.: Permo-Triassic Evaporites of the Polish Basin and Their Bearing on the Tectonic Evolution and Hydrocarbon System, an Overview, in: Permo-Triassic Salt Provinces of Europe, North Africa and the Atlantic Margins, edited by: Soto, J. I., Flinch, J. F., and Tari, G., Elsevier, Amsterdam, the Netherlands, 243–261, https://doi.org/10.1016/B978-0-12-809417-4.00012-4, 2017c.
Krzywiec, P., Stachowska, A., and Stypa, A.: The only way is up – On Mesozoic uplifts and basin inversion events in SE Poland, Geol. Soc. Spec. Publ., 469, 33–57, https://doi.org/10.1144/SP469.14, 2018.
Krzywiec, P., Kiersnowski, H., and Peryt, T. M.: Fault-controlled Permian (Rotliegend and Zechstein) sedimentation in central Poland Basin (Bydgoszcz – Szubin area) – insight from well and seismic data, German Journal of Geology [Zeitschrift der Deutschen Gesellschaft für Geowissenschaften], 170, 255–272, https://doi.org/10.1127/zdgg/2019/0198, 2019.
Krzywiec, P., Słonka, Ł., Nguyen, Q., Malinowski, M., Kufrasa, M., Stachowska, A., Huebscher, C., and Kramarska, R.: Late Cretaceous – Cenozoic history of the transition zone between the East European Craton and the Paleozoic Platform, Polish sector of the Baltic Sea, revealed by new offshore regional seismic data, EGU General Assembly, Vienna, Austria, 19–30 April 2021, EGU21-13383, https://doi.org/10.5194/egusphere-egu21-13383, 2021.
Kuberska, M., Kozłowska, A., Maliszewska, A., and Buniak, A.: Evolution of pore space in the Upper Carboniferous and Lower Permian sandstones from Western Pomerania, Przegląd Geol., 55, 853–860, 2007 (in Polish with English summary).
Kufrasa, M., Krzywiec, P., Gągała, Ł., Mazur, S., and Mikołajczak, M.: Geometry and kinematics of an incipient fold-and-thrust belt from geophysical data (Lublin Basin, Poland), J. Struct. Geol., 141, 104211, https://doi.org/10.1016/j.jsg.2020.104211, 2020.
Lamarche, J., Mansy, J.-M., Bergerat, F., Averbuch, O., Hakenberg, M., Lewandowski, M., Stupnicka, E., Świdrowska, J., Wajsprych, B., and Wieczorek, J.: Variscan tectonics in the Holy Cross Mountains (Poland) and the role of structural inheritance during Alpine tectonics, Tectonophysics, 313, 171–186, https://doi.org/10.1016/S0040-1951(99)00195-X, 1999.
Lassen, A., Thybo, H., and Berthelsen, A.: Reflection seismic evidence for Caledonian deformed sediments above Sveconorwegian basement in the southwestern Baltic Sea, Tectonics, 20, 268–276, https://doi.org/10.1029/2000TC900028, 2001.
Laurent, A., Averbuch, O., Beccaletto, L., Graveleau, F., Lacquement, F., Capar, L., and Marc, S.: 3-D structure of the Variscan Thrust Front in northern France: new insights from seismic reflection profiles, Tectonics, 40, e2020TC006642, https://doi.org/10.1029/2020TC006642, 2021.
Lazauskienė, J., Stephenson, R., Šliaupa, S., and van Wees, J.-D.: 3-D flexural modelling of the Silurian Baltic Basin, Tectonophysics, 346, 115–135, https://doi.org/10.1016/S0040-1951(01)00231-1, 2002.
Leszczyński, K.: Late Cretaceous inversion and salt tectonics in the Koszalin-Chojnice and Drawno-Człopa-Szamotuły zones, Pomeranian sector of the Mid-Polish Trough, Geol. Q., 46, 347–362, 2002.
Leszczyński, K.: The internal geometry and lithofacies pattern of the Upper Cretaceous-Danian sequence in the Polish Lowlands, Geol. Q., 56, 363–386, https://doi.org/10.7306/gq.1028, 2012.
Leveridge, B. E. and Hartley, A. J.: The Variscan Orogeny: The development and deformation of Devonian/ Carboniferous basins in: SW England and South Wales, in: The Geology of England and Wales, edited by: Brenchley, P. J., and Rawson, P. F., The Geological Society of London, London, UK, 225–255, https://doi.org/10.1144/GOEWP.10, 2006.
Lipiec, M. and Matyja, H.: Depositional architecture of the lower Carboniferous Sedimentary Basin in Pomerania, Pr. Państwowego Inst. Geol., 165, 101–112, 1998 (in Polish with English summary).
Littke, R., Bayer, U., Gajewski, D., and Nelskamp, S. (Eds.): Dynamics of Complex Intracontinental Basins: The Central European Basin System, Springer-Verlag, Berlin, Heidelberg, Germany, 519 pp., https://doi.org/10.1007/978-3-540-85085-4, 2008.
Lohr, T., Krawczyk, C. M., Tanner, D. C., Samiee, R., Endres, H., Oncken, O., Trappe, H., and Kukla, P. A.: Strain partitioning due to salt: Insights from interpretation of a 3D seismic data set in the NW German Basin, Basin Res., 19, 579–597, https://doi.org/10.1111/j.1365-2117.2007.00338.x, 2007.
Maliszewska, A., Jackowicz, E., Kuberska, M., and Kiersnowski, H.: Lower Permian (Rotliegend) rocks of western Poland – A petrographic monograph, Pr. Państwowego Inst. Geol., 204, 6–115, 2016 (in Polish with English summary).
Marsh, N., Imber, J., Holdsworth, R. E., Brockbank, P., and Ringrose, P.: The structural evolution of the Halten Terrace, offshore Mid-Norway: Extensional fault growth and strain localisation in a multi-layer brittle-ductile system, Basin Res., 22, 195–214, https://doi.org/10.1111/j.1365-2117.2009.00404.x, 2010.
Martínez, F., Arriagada, C., Mpodozis, C., and Peña, M.: The Lautaro Basin: A record of inversion tectonics in northern Chile, Andean Geology, 39, 258–278, https://doi.org/10.5027/andgeoV39n2-a04, 2012.
Martínez Catalán, J. R., Collett, S., Schulmann, K., Aleksandrowski, P., and Mazur, S.: Correlation of allochthonous terranes and major tectonostratigraphic domains between NW Iberia and the Bohemian Massif, European Variscan belt, Int. J. Earth Sci., 109, 1105–1131, https://doi.org/10.1007/s00531-019-01800-z, 2020.
Matyja, H.: Upper Devonian of western Pomerania, Acta Geol. Pol., 43, 27–94, 1993 (in Polish with English summary).
Matyja, H.: Depositional architecture of the Devonian basin in the Pomorze-Kujawy area, Pr. Państwowego Inst. Geol., 165, 73–88, 1998 (in Polish with English summary).
Matyja, H.: Stratigraphy and facies development of Devonian and Carboniferous deposits in the Pomeranian Basin and in the western part of the Baltic Basin and palaeogeography of the northern TESZ during Late Palaeozoic times, Pr. Państwowego Inst. Geol., 186, 79–122, 2006 (in Polish with English summary).
Matyja, H.: Pomeranian basin (NW Poland) and its sedimentary evolution during Mississippian times, Geol. J., 43, 123–150, https://doi.org/10.1002/gj.1117, 2008.
Maynard, J. R., Hofmann, W., Dunay, R. E., Benthan, P. N., Dean, K. P., and Watson, I.: The Carboniferous of Western Europe: The development of a petroleum system, Petrol. Geosci., 3, 97–115, https://doi.org/10.1144/petgeo.3.2.97, 1997.
Maystrenko, Y., Stovba, S., Stephenson, R., Bayer, U., Menyoli, E., Gajewski, D., Huebscher, C., Rabbel, W., Saintot, A., Starostenko, V., Thybo, H., and Tolkunov, A.: Crustal-scale pop-up structure in cratonic lithosphere: DOBRE deep seismic reflection study of the Donbas fold belt, Ukraine, Geology, 31, 733–736, https://doi.org/10.1130/G19329.1, 2003.
Mazur, S., Scheck-Wenderoth, M., and Krzywiec, P.: Different modes of the Late Cretaceous–Early Tertiary inversion in the North German and Polish basins, Int. J. Earth Sci., 94, 782–798, https://doi.org/10.1007/s00531-005-0016-z, 2005.
Mazur, S., Aleksandrowski, P., Turniak, K., Krzemiński, L., Mastalerz, K., Górecka-Nowak, A., Kurowski, L., Krzywiec, P., Żelaźniewicz, A., and Fanning, M. C.: Uplift and late orogenic deformation of the Central European Variscan belt as revealed by sediment provenance and structural record in the Carboniferous foreland basin of western Poland, Int. J. Earth Sci., 99, 47–64, https://doi.org/10.1007/s00531-008-0367-3, 2010.
Mazur, S., Mikołajczak, M., Krzywiec, P., Malinowski, M., Buffenmyer, V., and Lewandowski, M.: Is the Teisseyre-Tornquist Zone an ancient plate boundary of Baltica?, Tectonics, 34, 2465–2477, https://doi.org/10.1002/2015TC003934, 2015.
Mazur, S., Mikołajczak, M., Krzywiec, P., Malinowski, M., Lewandowski, M., and Buffenmyer, V.: Pomeranian Caledonides, NW Poland – A collisional suture or thin-skinned fold-and-thrust belt?, Tectonophysics, 692, 29–43, https://doi.org/10.1016/j.tecto.2016.06.017, 2016.
Mazur, S., Porębski, S. J., Kędzior, A., Paszkowski, M., Podhalańska, T., and Poprawa, P.: Refined timing and kinematics for Baltica-Avalonia convergence based on the sedimentary record of a foreland basin, Terra Nova, 30, 8–16, https://doi.org/10.1111/ter.12302, 2018.
Mazur, S., Aleksandrowski, P., Gągała, Ł., Krzywiec, P., Żaba, J., Gaidzik, K., and Sikora, R.: Late Palaeozoic strike-slip tectonics versus oroclinal bending at the SW outskirts of Baltica: Case of the Variscan belt's eastern end in Poland, Int. J. Earth Sci., 109, 1133–1160, https://doi.org/10.1007/s00531-019-01814-7, 2020.
Mazur, S., Malinowski, M., Maystrenko, Y. P., and Gągała, Ł.: Pre-existing lithospheric weak zone and its impact on continental rifting – The Mid-Polish Trough, Central European Basin System, Global Planet. Change, 198, 103417, https://doi.org/10.1016/j.gloplacha.2021.103417, 2021.
McCann, T.: The tectonosedimentary evolution of the northern margin of the Carboniferous foreland basin of NE Germany, Tectonophysics, 313, 119–144, https://doi.org/10.1016/S0040-1951(99)00193-6, 1999.
McCann, T., Pascal, C., Timmerman, M. J., Krzywiec, P., López-Gómez, J., Wetzel, L., Krawczyk, C. M., Rieke, H., and Lamarche, J.: Post-Variscan (end Carboniferous-Early Permian) basin evolution in Western and Central Europe, Geol. Soc. Lond. Mem., 32, 355–388, https://doi.org/10.1144/GSL.MEM.2006.032.01.22, 2006.
McCann, T., Skompski, S., Poty, E., Dusar, M., Vozarova, A., Schneider, J., Wetzel, A., Krainer, K., Kornpihl, K., Schafer, A., Krings, M., Oplustil, S., and Tait, J.: Carboniferous, in: The Geology of Central Europe Volume 1: Precambrian and Palaeozoic, edited by: McCann, T., The Geological Society of London, London, UK, 411–529, https://doi.org/10.1144/CEV1P.9, 2008.
McClay, K. R.: The geometries and kinematics of inverted fault systems: a review of analogue model studies, Geol. Soc. Lond. Spec. Publ., 88, 97–118, https://doi.org/10.1144/GSL.SP.1995.088.01.07, 1995.
McClay, K. R. and Scott, A. D.: Experimental models of hangingwall deformation in ramp-flat listric extensional fault systems, Tectonophysics, 188, 85–96, https://doi.org/10.1016/0040-1951(91)90316-K, 1991.
Meissner, R., Thybo, H., and Abramovitz, T.: Interwedging and inversion structures around the trans-European suture zone in the Baltic Sea, a manifestation of compressive tectonic phases, Tectonophysics, 360, 265–280, https://doi.org/10.1016/S0040-1951(02)00356-6, 2002.
Minguely, B., Averbuch, O., Patin, M., Rolin, D., Hanot, F., and Bergerat, F.: Inversion tectonics at the northern margin of the Paris Basin (northern France): New evidence from seismic profiles and boreholes interpolation in the Artois area, B. Soc. Geol. Fr., 181, 429–442, https://doi.org/10.2113/gssgfbull.181.5.429, 2010.
Mitra, S. and Islam, Q. T.: Experimental (clay) models of inversion structures, Tectonophysics, 230, 211–222, https://doi.org/10.1016/0040-1951(94)90136-8, 1994.
Muszyński, A., Biernacka, J., Lorenc, S., Protas, A., Urbanek, Z., and Wojewoda, J.: Petrology and depositional environment of Lower Carboniferous rocks near Dygowo and Kłanino (the Koszalin-Chojnice Zone), Geologos, 1, 93–126, 1996 (in Polish with English summary).
Narkiewicz, M.: Development and inversion of Devonian and Carboniferous basins in the eastern part of the Variscan foreland (Poland), Geol. Q., 51, 231–256, 2007.
Narkiewicz, M.: The Variscan foreland in Poland revisited: New data and new concepts, Geol. Q., 64, 377–401, https://doi.org/10.7306/gq.1511, 2020.
Narkiewicz, M., Poprawa, P., Lipiec, M., Matyja, H., and Miłaczewski, L.: Paleogeographic and tectonic setting and the Devonian-Carboniferous subsidence development of the Pomerania and Radom-Lublin regions, Pr. Państwowego Inst. Geol., 165, 31–49, 1998 (in Polish with English summary).
Norton, M. G., McClay, K. R., and Way, N. A.: Tectonic evolution of Devonian basins in northern Scotland and southern Norway, Norsk Geol. Tidsskr., 67, 323–338, 1987.
Okay, A. I. and Topuz, G.: Variscan orogeny in the Black Sea region, Int. J. Earth Sci., 106, 569–592, https://doi.org/10.1007/s00531-016-1395-z, 2017.
Oncken, O., von Winterfeld, C., and Dittmar, U.: Accretion of a rifted passive margin: The Late Paleozoic Rhenohercynian fold and thrust belt (Middle European Variscides), Tectonics, 18, 75–91, https://doi.org/10.1029/98TC02763, 1999.
Opluštil, S. and Cleal, C. J.: A comparative analysis of some Late Carboniferous basins of Variscan Europe, Geol. Mag., 144, 417–448, https://doi.org/10.1017/S0016756807003330, 2007.
Osmundsen, P. T. and Andersen, T. B.: The middle Devonian basins of western Norway: sedimentary response to large-scale transtensional tectonics? Tectonophysics, 332, 51–68, https://doi.org/10.1016/S0040-1951(00)00249-3, 2001.
Panien, M., Schreurs, G., and Pfiffner, A.: Sandbox experiments on basin inversion: Testing the influence of basin orientation and basin fill, J. Struct. Geol., 27, 433–445, https://doi.org/10.1016/j.jsg.2004.11.001, 2005.
Panien, M., Buiter, S., Schreurs, G., and Pfiffner, O.-A.: Inversion of a symmetric basin: insights from a comparison between analogue and numerical experiments, in: Analogue and Numerical Modelling of Crustal Scale Processes, edited by: Buiter, S. J. H. and Schreurs, G., Geological Society, London, UK, Geol. Soc. Spec. Publ., 253, 253–270, https://doi.org/10.1144/gsl.sp.2006.253.01.13, 2006.
Peace, G. R. and Besly, B. M.: End-Carboniferous fold-thrust structures, Oxfordshire, UK: Implications for the structural evolution of the late Variscan foreland of south-central England, J. Geol. Soc. Lond., 154, 225–237, https://doi.org/10.1144/gsjgs.154.2.0225, 1997.
Pharaoh, T. C., Dusar, M., Geluk, M. C., Kockel, F., Krawczyk, C. M., Krzywiec, P., Scheck-Wenderoth, M., Thybo, H., Vejbæk, O. V., and van Wees, J. D.: Tectonic evolution, in: Petroleum Geological Atlas of the Southern Permian Basin Area, edited by: Doornenbal, H. and Stevenson, A., EAGE Publications BV, Houten, the Netherlands, 25–57, ISBN 978-90-73781-61-0, 2010.
Pharaoh, T. C., Haslam, R., Hough, E., Kirk, K., Leslie, G., Schofield, D., and Heafford, A.: The Môn–Deemster–Ribblesdale fold–thrust belt, central UK: A concealed Variscan inversion belt located on weak Caledonian crust, Geol. Soc. Lond. Spec. Publ., 490, 153–176, https://doi.org/10.1144/SP490-2018-109, 2020.
Piwocki, M. and Kramarska, R.: Polish Lowlands and their southern rim – basics of stratigraphy, in: Geological Setting of Poland, Vol. 1, Stratigraphy, Part 3a, Cenozoic, Paleogene, Neogene, edited by: Peryt, T. M. and Piwocki, M., Państwowy Instytut Geologiczny, Warszawa, Poland, 19–22, 2004 (in Polish with English summary).
Podhalańska, T. and Modliński, Z.: Stratigraphy and facies characteristics of the Ordovician and Silurian deposits of the Koszalin-Chojnice Zone: Similarities and differences to the western margin of the East European Craton and the Rügen area, Pr. Państwowego Inst. Geol., 186, 39–78, 2006 (in Polish with English summary).
Polish Geological Institute: Central Geological Database and National Geological Archive, http://geoportal.pgi.gov.pl, last access: 16 March 2022.
Poprawa, P.: Development of the Caledonian collision zone along the western margin of Baltica and its relation to the foreland basin, Pr. Państwowego Inst. Geol., 186, 189–214, 2006 (in Polish with English summary).
Poprawa, P.: Geological setting and Ediacaran–Palaeozoic evolution of the western slope of the East European Craton and adjacent regions, Ann. Soc. Geol. Pol., 89, 347–380, https://doi.org/10.14241/asgp.2019.23, 2019.
Poprawa, P., Šliaupa, S., Stephenson, R., and Lazauskiene, J.: Late Vendian–Early Palæozoic tectonic evolution of the Baltic Basin: Regional tectonic implications from subsidence analysis, Tectonophysics, 314, 219–239, https://doi.org/10.1016/S0040-1951(99)00245-0, 1999.
Poprawa, P., Krzemińska, E., Pacześna, J., and Amstrong, R.: Geochronology of the Volyn volcanic complex at the western slope of the East European Craton – Relevance to the Neoproterozoic rifting and the break-up of Rodinia/Pannotia, Precambrian Res., 346, 105817, https://doi.org/10.1016/j.precamres.2020.105817, 2020.
Powell, C. M. and Williams, G. D.: The Lewis Thrust/Rocky Mountain trench fault system in Northwest Montana, USA: an example of negative inversion tectonics?, Geol. Soc. Lond. Spec. Publ., 44, 223–234, 1989.
Resak, M., Narkiewicz, M., and Littke, R.: New basin modelling results from the Polish part of the Central European Basin system: implications for the Late Cretaceous–Early Paleogene structural inversion, Int. J. Earth Sci., 97, 955–972, https://doi.org/10.1007/s00531-007-0246-3, 2008.
Rowan, M. G. and Jarvie, A.: Crustal extension and salt tectonics of the Danmarkshavn Ridge and adjacent basins, NE Greenland, Mar. Petrol. Geol., 117, 104339, https://doi.org/10.1016/j.marpetgeo.2020.104339, 2020.
Rowan, M. and Krzywiec, P.: The Szamotuły salt diapir and Mid-Polish Trough: Decoupling during both Triassic-Jurassic rifting and Alpine inversion – Interpretation, 2, SM1–SM18, https://doi.org/10.1190/INT-2014-0028.1, 2014.
Scheck-Wenderoth, M., Krzywiec, P., Zuhlke, R., Maystrenko, Y., and Froitzheim, N.: Permian to Cretaceous tectonics, in: The Geology of Central Europe Volume 2: Mesozoic and Cenozoic, edited by: McCann, T., The Geological Society of London, London, 999–1030, https://doi.org/10.1144/CEV2P.4, 2008.
Schori, M., Zwaan, F., Schreurs, G., and Mosar, J.: Pre-existing Basement Faults Controlling Deformation in the Jura Mountains Fold-and-Thrust Belt: Insights from Analogue Models, Tectonophysics, 814, 228980, https://doi.org/10.1016/j.tecto.2021.228980, 2021.
Scisciani, V.: Styles of positive inversion tectonics in the Central Apennines and in the Adriatic foreland: Implications for the evolution of the Apennine chain (Italy), J. Struct. Geol., 31, 1276–1294, https://doi.org/10.1016/j.jsg.2009.02.004, 2009.
Scisciani, V., Patruno, S., D'Intino, N., and Esestime, P.: Paleozoic basin reactivation and inversion of the underexplored northern North Sea platforms: a cross-border approach. Geol. Soc. Lond. Spec. Publ., 494, SP494-2020-252, https://doi.org/10.1144/SP494-2020-252, 2021.
Seidel, E., Meschede, M., and Obst, K.: The Wiek Fault System east of Rügen Island: origin, tectonic phases and its relationship to the Trans-European Suture Zone, Geol. Soc. Lond. Spec. Publ., 469, 59–82, https://doi.org/10.1144/SP469.10, 2018.
Séranne, M., Chauvet, A., Seguret, M., and Brunel, M.: Tectonics of the Devonian collapse-basins of western Norway, B. Soc. Geol. Fr., 5, 489–499, https://doi.org/10.2113/gssgfbull.V.3.489, 1989.
Shail, R. K. and Leveridge, B. E.: The Rhenohercynian passive margin of SW England: Development, inversion and extensional reactivation, C.R. Geosci., 341, 140–155, https://doi.org/10.1016/j.crte.2008.11.002, 2009.
Smit, J., van Wees, J.-D., and Cloetingh, S.: Early Carboniferous extension in East Avalonia: 350 My record of lithospheric memory, Mar. Petrol. Geol., 92, 1010–1027, https://doi.org/10.1016/j.marpetgeo.2018.01.004, 2018.
Smith, N.: Variscan inversion within the Cheshire Basin, England: Carboniferous evolution north of the Variscan Front, Tectonophysics, 309, 211–225, https://doi.org/10.1016/S0040-1951(99)00140-7, 1999.
Sopher, D., Erlström, M., Bell, N., and Juhlin, C.: The structure and stratigraphy of the sedimentary succession in the Swedish sector of the Baltic Basin: New insights from vintage 2D marine seismic data, Tectonophysics, 676, 90–111, https://doi.org/10.1016/j.tecto.2016.03.012, 2016.
Soto, R., Casas-Sainz, A. M., and Del Río, P.: Geometry of half-grabens containing a mid-level viscous décollement, Basin Res., 19, 437–450, https://doi.org/10.1111/j.1365-2117.2007.00328.x, 2007.
Stemmerik, L.: Late Palaeozoic evolution of the north Atlantic margin of Pangea. Palaeogeogr. Palaeocl., 161, 95–126, https://doi.org/10.1016/S0031-0182(00)00119-X, 2000.
Stephenson, R. A., Narkiewicz, M., Dadlez, R., van Wees, J.-D., and Andriessen, P.: Tectonic subsidence modelling of the Polish Basin in the light of new data on crustal structure and magnitude of inversion, Sediment. Geol., 156, 59–70, https://doi.org/10.1016/S0037-0738(02)00282-8, 2003.
Stewart, S. A.: Geometry of thin-skinned tectonic systems in relation to detachment layer thickness in sedimentary basins, Tectonics, 18, 719–732, https://doi.org/10.1029/1999TC900018, 1999.
Tari, G., Arbouille, D., Schléder, Z., and Tóth, T.: Inversion tectonics: a brief petroleum industry perspective, Solid Earth, 11, 1865–1889, https://doi.org/10.5194/se-11-1865-2020, 2020.
Tari, G., Bada, G., Beidinger, A., Csizmeg, J., Danišik, M., Gjerazi, I., Grasemann, B., Kováč, M., Plašienka, D., Šujan, M., and Szafián, P.: The connection between the Alps and the Carpathians beneath the Pannonian Basin: Selective reactivation of Alpine nappe contacts during Miocene extension, Global Planet. Change, 197, 103401, https://doi.org/10.1016/j.gloplacha.2020.103401, 2021.
Tavarnelli, E.: Normal faults in thrust sheets: pre-orogenic extension, post-orogenic extension, or both?, J. Struct. Geol., 21, 1011–1018, 1999.
Tomaszczyk, M. and Jarosiński, M.: The Kock Fault Zone as an indicator of tectonic stress regime changes at the margin of the East European Craton (Poland), Geol. Q., 61, 908–925, https://doi.org/10.7306/gq.1380, 2017.
Tomek, F., Vacek, F., Žák, J., Petronis, M. S., Verner, K., and Foucher, M. S.: Polykinematic foreland basins initiated during orthogonal convergence and terminated by orogen-oblique strike-slip faulting: An example from the northeastern Variscan belt, Tectonophysics, 766, 379–397, https://doi.org/10.1016/j.tecto.2019.05.023, 2019.
Tortorici, G., Romagnoli, G., Grassi, S., Imposa, S., Lombardo, G., Panzera, F., and Catalano, S.: Quaternary negative tectonic inversion along the Sibillini Mts. thrust zone: the Arquata del Tronto case history (Central Italy), Environ. Earth Sci., 78, 37, https://doi.org/10.1007/s12665-018-8021-2, 2019.
Trela, M., Kasprzyk, M., and Saj, A.: Opracowanie badań sejsmicznych 3D dla tematów Daszewo N i Białogard, unpublished report, Geofizyka Toruń S.A., 2011.
van Wees, J.-D., Stephenson, R., Ziegler, P., Bayer, U., McCann, T., Dadlez, R., Gaupp, R., Narkiewicz, M., Bitzer, F., and Scheck, M.: On the origin of the Southern Permian Basin, Central Europe, Mar. Petrol. Geol., 17, 43–59, https://doi.org/10.1016/S0264-8172(99)00052-5, 2000.
Velasco, M. S., Bennett, R. A., Johnson, R. A., and Hreinsdóttir, S.: Subsurface fault geometries and crustal extension in the eastern Basin and Range Province, western U.S., Tectonophysics, 488, 131–142, https://doi.org/10.1016/j.tecto.2009.05.010, 2010.
Voigt, T., Kley, J., and Voigt, S.: Dawn and dusk of Late Cretaceous basin inversion in central Europe, Solid Earth, 12, 1443–1471, https://doi.org/10.5194/se-12-1443-2021, 2021.
von Hartmann, H.: Deformation of the Carboniferous on the Oldenburg High and the Location of the Variscan Front in Northwest Germany, Neth. J. Geosci., 82, 169–176, https://doi.org/10.1017/S0016774600020722, 2003.
Wagner, R.: Stratigraphy and evolution of the Zechstein basin in the Polish Lowlands, Pr. Państwowego Inst. Geol., 146, 1–71, 1994 (in Polish with English summary).
Wagner, R.: Zechstein, in: Paleogeographic atlas of epicontinental Permian and Mesozoic in Poland, 1 : 2 500 000, edited by: Dadlez, R., Marek, S., and Pokorski, J., Państwowy Instytut Geologiczny, Warszawa, Poland, 1998.
Warr, L. N.: The Variscan Orogeny: The Welding of Pangaea, in: Geological History of Britain and Ireland, edited by: Woodcock, N. H. and Strachan, R. A., John Wiley & Sons, Ltd, Chichester, UK, 274–298, https://doi.org/10.1002/9781118274064.ch15, 2012.
Warsitzka, M., Závada, P., Jähne-Klingberg, F., and Krzywiec, P.: Contribution of gravity gliding in salt-bearing rift basins – a new experimental setup for simulating salt tectonics under the influence of sub-salt extension and tilting, Solid Earth, 12, 1987–2020, https://doi.org/10.5194/se-12-1987-2021, 2021.
Williams, G. D., Powell, C. M., and Cooper, M. A.: Geometry and kinematics of inversion tectonics, Geol. Soc. Lond. Spec. Publ., 44, 3–15, https://doi.org/10.1144/GSL.SP.1989.044.01.02, 1989.
Withjack, M. O. and Callaway, S.: Active normal faulting beneath a salt layer: An experimental study of deformation patterns in the cover sequence, Am. Assoc. Petr. Geol. B., 84, 627–651, https://doi.org/10.1306/c9ebce73-1735-11d7-8645000102c1865d, 2000.
Withjack, M. O., Baum, M. S., and Schlische, R. W.: Influence of preexisting fault fabric on inversion-related deformation: A case study of the inverted Fundy rift basin, southeastern Canada, Tectonics, 29, TC6004, https://doi.org/10.1029/2010TC002744, 2010.
Xiao, H. and Suppe, J.: Origin of rollover, Am. Assoc. Petr. Geol. B., 76, 509–529, https://doi.org/10.1306/bdff8858-1718-11d7-8645000102c1865d, 1992.
Zayats, K.: The structure of minerals of the Western region of Ukraine on the basis of seismic studies and the directions for oil and gas exploration studies (in Ukrainian), USGEI, Lviv, Ukraine, ISBN 978-966-464-003-6, 2015.
Ziegler, P. A.: Geological Atlas of Western and Central Europe, Shell Internationale Petroleum Maatschappij B.V. and Geological Society Publishing House, Bath, UK, ISBN 906-6-4412-59, 978-90-66441-255, 1990.
Ziegler, P. A., Bertotti, G., and Cloetingh, S.: Dynamic processes controlling foreland development – The role of mechanical (de)coupling of orogenic wedges and forelands, Stephan Mueller Spec. Publ. Ser., 1, 17–56, https://doi.org/10.5194/smsps-1-17-2002, 2002.
Znosko, J.: The problem of Caledonides and the border of Precambrian platform in Poland, Biul. Inst. Geol., 188, 5–72, 1965 (in Polish).
Żaba, J. and Poprawa, P.: Deformation history of the Koszalin-Chojnice zone (Pomeranian segment of TESZ, NW Poland) – constraints from structural analysis of Palaeozoic and Mesozoic successions in Polskie Łąki PIG-1 and Toruń-1 boreholes, Pr. Państwowego Inst. Geol., 186, 225–252, 2006.
Żelichowski, A. M.: Lithostratigraphy and sedimentological-paleogeographical development in Western Pomerania, Pr. Państwowego Inst. Geol., 148, 97–100, 1995.