the Creative Commons Attribution 4.0 License.
the Creative Commons Attribution 4.0 License.
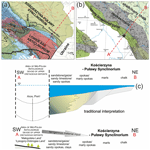
Deep vs. shallow – two contrasting theories? A tectonically activated Late Cretaceous deltaic system in the axial part of the Mid-Polish Trough: a case study from southeast Poland
Zbyszek Remin
Michał Cyglicki
Mariusz Niechwedowicz
The Polish Basin is a part of a trans-European sedimentary basin comprising the Central European Basin System and stretching from Denmark through Germany to southeastern Poland, and even further to the south into Ukraine. It experienced uplift during the Late Cretaceous, which consequently resulted in the inversion of its axial part (i.e., the Mid-Polish Trough) and development into the Mid-Polish Anticlinorium. In many existing paleotectonic interpretations, southeast Poland – i.e., the subsurface San Anticlinorium and the present-day Roztocze Hills – was included in the Mid-Polish Trough during the Late Cretaceous, representing its axial, most subsiding part. This paleotectonic model was the basis for facies and bathymetric interpretations that assumed Upper Cretaceous sediments, deposited close to the axial part of the Mid-Polish Trough (e.g., in the Roztocze Hills), constituted the deepest facies. In recent years, several studies have contradicted this notion. A growing body of data suggests that this area (southeast Poland) was already a landmass by the Coniacian and Santonian – and certainly in the Campanian and Maastrichtian – rather than forming the deepest part of the Polish Basin.
The shallow marginal marine, cyclic middle Campanian, marly to sandy deposits, recently discovered in the Roztocze Hills, were interpreted to be of deltaic origin. This has led to the adoption of new facies and bathymetric models, which – notably – are in stark opposition to most previous interpretations. Notably, the new interpretation implies the presence of a landmass in the place where, in prior frameworks, the deepest and most subsiding part of the Polish Basin (i.e., Mid-Polish Trough) was located during the Cretaceous.
Here, we document this Late Cretaceous depositional system – the Szozdy delta developed in the axial part of the Mid-Polish Trough. These middle Campanian deposits crop out extensively in the Roztocze Hills region, close to the village of Szozdy, and exhibit coarsening-upward tripartite cyclothems. Three facies associations have been distinguished: (1) dark grey calcareous mudstones, (2) a yellow calcareous sandstone, and (3) calcareous gaize lithofacies. The sequence, as a whole, was accumulated via the repeated progradation and abandonment of deltaic complexes on the delta front platform setting, with the primary transport direction originating from the axis of the inverting Mid-Polish Trough (thus from the subsurface San Anticlinorium) toward the northeast. This interpretation is supported by a suite of sedimentological, palynofacies, and heavy mineral data.
The development of the Szozdy delta system is framed in the context of the dynamic tectonic processes operating contemporaneously in southeast Poland: that is, tectonic inversion (uplift) on one hand, and the generation of new accommodation space via enhanced subsidence on the other. This discovery sheds new light on our understanding of Late Cretaceous facies distribution, bathymetry, and paleogeography and might potentially suggest a different burial history than assumed so far.
- Article
(12303 KB) - Full-text XML
- BibTeX
- EndNote
During the Late Cretaceous, central and western Europe has been affected by compressional tectonics, which resulted in deformations observed across the whole Central European Basin System. This tectonic event induced the uplift and inversion of the basement units. The uplifted structures were eroded and material were redeposited in a newly formed flexural basin (marginal troughs) (e.g., Ziegler, 1990; Nielsen and Hansen, 2000; Krzywiec, 2006, Krzywiec and Stachowska, 2016; Kley and Voigt, 2008; Voigt et al., 2021). Due to Late Cretaceous inversion tectonics, the paleogeographic and facies architecture of the Central European Basin System were markedly rebuilt especially in a belt along the margin of the East European Platform (Fig. 1a).
The Polish Basin, as part of a large, Mesozoic trans-European sedimentary basin, underwent similar tectonic stress (inversion tectonics) to the whole Central European Basin System (for review compare: Krzywiec, 2002; Krzywiec et al., 2009, 2018). The Late Cretaceous paleotectonic evolution of the Polish Basin has been of considerable interest to geologists for more than a hundred years. The tectonic evolution and timing of the final tectonic inversion of the Mid-Polish Trough, especially its southeastern segment (Figs. 1b and 2a), is still a matter of active study, with no clear consensus reached to date (see reviews in Krzywiec et al., 2009; Walaszczyk and Remin, 2015; Krzywiec et al., 2018). The most important conclusion reached from these debates is that existing models developed over the past 50 years do not and cannot explain the entire geological history of this fragment of Poland (a part of the supposed Mid-Polish Trough), during the Mesozoic and, especially, the Late Cretaceous.
Over the past few decades, two independent paleotectonic models have been developed, focused mainly on the onset (and timing) of the inversion (uplift) of the Mid-Polish Trough (including its southeastern segment), and the consequent implications for facies and bathymetric (environmental) interpretations. Ultimately, the inversion-related uplift and subsequent erosion of the former axial part of the basin led to the formation of a prominent structural unit – the Mid-Polish Anticlinorium (Figs. 1b and 2a) (for reviews see Krzywiec et al., 2009; Walaszczyk and Remin, 2015; Krzywiec et al., 2018) with two synclinoria (troughs) bordering the Mid-Polish Anticlinorium toward NE (Kościerzyna-Puławy Synclinorium) and SW (Szczecin-Łódź-Miechów Synclinorium). The surrounding troughs (synclinoria) were formed by the mechanism initially proposed by Nielsen and Hansen (2000) for other inverted basins across Europe.
According to the more traditional and so far widely accepted interpretations, the southeastern edge of the Mid-Polish Trough (an axial part of the Polish Basin) (Fig. 2a), which is currently almost devoid of Mesozoic overburden (Fig. 2a) – represented its axial part and contained the deepest, most subsiding sedimentary environments during the Late Cretaceous (e.g., Kutek and Głazek, 1972; Hakenberg and Świdrowska, 1998, 2001; Świdrowska and Hakenberg, 1999; Świdrowska, 2007; Świdrowska et al., 2008). In turn, this paleotectonic model formed the basis for facies and bathymetric interpretations that largely suggest sediments deposited close to the axial part of the Polish Basin (i.e., the Mid-Polish Trough), like those in the Roztocze Hills (southeast Poland) would constitute the deepest facies (Fig. 2c).
A series of studies performed over the past few years have supplied data in stark contradiction to the above interpretation, showing that already by the Coniacian and Santonian (and possibly even by the Late Turonian) the axial part of the Polish Basin (i.e., the Mid-Polish Trough) should be considered an emergent landmass or paleomorphological barrier, rather than as the deepest part of the basin, especially in southeastern Poland (e.g., Krzywiec et al., 2009; Remin et al., 2015a; Walaszczyk and Remin, 2015; Remin et al., 2016; Krzywiec et al., 2018; Remin, 2018). This view is supported by the presence of clearly shallow-water facies along the northeastern edge of the present-day Mid-Polish Anticlinorium, especially in southeastern Poland, thus in the area where deepest facies should be expected according to the model of Kutek and Głazek (1972): these are the shallow-water Turonian Janików Limestone and deltaically influenced sedimentation of the Roztocze Hills formed during the Campanian and Maastrichtian (Remin et al., 2015a; Walaszczyk and Remin, 2015). Additionally, seismic data show progradational bodies trending from the SW toward the NE (Krzywiec et al., 2009, 2018), thus extending from the supposedly axial part of the basin toward the northeast. However, all these data did not provide definitive proof for the existence of an emerged landmass in southeast Poland during the Late Cretaceous, and when treated individually their value and insights might be underestimated.
Remin et al. (2015a) provided a conceptual interpretation that the middle Campanian and ?Maastrichtian siliciclastic deposits of the Roztocze Hills were of deltaic origin with the main source area located to the SW: this in a place where, according to the widely accepted paleotectonic model of Kutek and Głazek (1972) and several later authors, the axial and deepest part of the Mid-Polish Trough was located (Fig. 2c). The implementation of the new concept suggested a need for a dramatic revision to the existing paleotectonic model of the southeastern part of the Polish Basin and drove the adoption of a new facies and bathymetric model for several Late Cretaceous facies, one that was essentially the opposite of most previous interpretations (compare Fig. 2c and d and discussion in Krzywiec et al., 2009; Remin et al., 2015a; Walaszczyk and Remin, 2015; Krzywiec et al., 2018). Since this theoretical interpretation lacked precise stratigraphically and sedimentologically grounded corroboration, the present paper fills this gap by providing evidence to a wider audience that the development of the Szozdy deltaic system was induced by active inversion tectonics.
The study area in the Roztocze Hills gives direct insight into this special Campanian and Maastrichtian sedimentary succession, thus allowing for precise bio- and chronostratigraphic dating, essential for detailed sedimentological, facies, and paleotectonic interpretations.
These new data sets provide the foundation for the first direct sedimentological, petrographic, and palynofacies evidence for the presence of an emerged landmass in an area now devoid of Mesozoic cover – that is, the subsurface San Anticlinorium (the SE part of the Mid-Polish Anticlinorium; Fig. 1b) – which had previously been considered to be deeply submerged during the Late Cretaceous. These data at least in part confirm the earlier concepts of, for example, Krzywiec et al. (2009, 2018) based on subsurface seismic data.
The objective of this paper is to show how a dynamic tectonic regime, including spatially variable inversion processes (uplift) and rapid subsidence, coupled with a possible wave and/or bottom current transport processes and significant terrigenous sediment input, drove the development of the Szozdy deltaic system. Its subaquatic remnants, originally developed in the axial part of the Mid-Polish Trough, are preserved in the rock record of the Roztocze Hills.
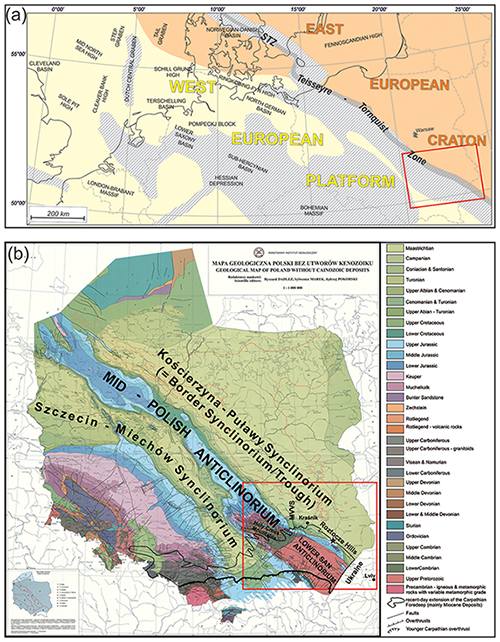
Figure 1(a) Regional schematic map of western and central Europe (after Krzywiec et al., 2018, with further references) with the schematic extent of the Late Cretaceous–Paleocene inversion (patterned area); red rectangle – area shown in (b) and in Fig. 2; (b) general position of the Roztocze Hills and San Anticlinorium in the geological map of Poland excluding Cenozoic deposits, adopted from Dadlez et al. (2000) – except for the recent-day extension of the Carpathian Foredeep; MVVS: Middle Vistula Valley Section.
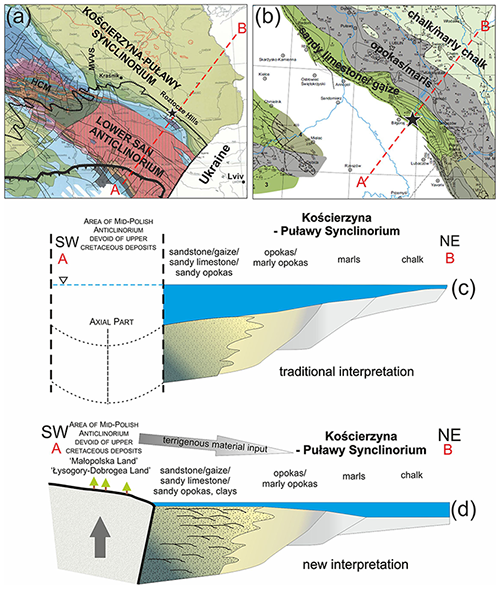
Figure 2(a) A close-up view of the Roztocze Hills area, relative to the position of the Holy Cross Mountains and Lower San Anticlinorium; (b) the general distribution of Campanian lithofacies in southeast Poland (adopted from Świdrowska, 2007) – sandy limestone: deep; chalk: shallow; red dashed line – an interpreted cross section of two competing tectonic concepts (c) and (d); (c) a facies and bathymetric sedimentation model based on traditional interpretations, assuming the deep-water character of deposits close to the axial part of the Mid-Polish Trough; vertical dashed-lines indicate areas devoid of Cretaceous deposits at present; (d) an alternative depositional, structural, and environmental interpretation of facies and bathymetry in a cross section perpendicular to the axis of the Mid-Polish Anticlinorium (adopted from Remin et al., 2015a; Walaszczyk and Remin, 2015).
Broadly speaking, the current literature hosts two theories regarding the onset of Mid-Polish Trough inversion tectonics and, subsequently, the formation of the Mid-Polish Anticlinorium (for a thorough review, see Krzywiec et al., 2009; Walaszczyk and Remin, 2015; Krzywiec et al., 2018).
The first – and more widely accepted, at least historically – concept holds that the inversion of the Mid-Polish Trough began during the Late Maastrichtian and Paleogene (e.g., Kutek and Głazek, 1972; Hakenberg and Świdrowska, 1998, 2001; Świdrowska and Hakenberg, 1999; Świdrowska, 2007; Świdrowska et al., 2008). Kutek and Głazek`s (1972) paper was indisputably a benchmark for interpretations of the Late Cretaceous paleogeographic and paleotectonic evolution of the southeastern segment of the Polish Basin, and especially its axial part – the Mid-Polish Trough.
Kutek and Głazek (1972) provided palinspastic restoration of the paleothickness of particular stratigraphic units on transects across the Mid-Polish Anticlinorium in the Holy Cross Mountains (to the NW from the present study area – compare Figs. 1b and 2a). In their framework, the increase in thickness of Mesozoic sedimentary successions toward the present-day Mid-Polish Anticlinorium (the area devoid of Mesozoic cover) (Figs. 1b and 2a) constituted the key argument for the highest burial and the presence of a depocenter in the axial part of the Mid-Polish Trough, at least until the early Maastrichtian. This also implies that the area currently devoid of Mesozoic remnants was deeply buried by those deposits.
The general framework of Kutek and Głazek (1972) was widely accepted, and for decades dominated thinking about the Mesozoic paleotectonic evolution of the Polish Basin. In their model, the southeastern part of the Polish Basin, the Mid-Polish Trough, would represent the axial, most subsiding part of the basin during the Late Cretaceous (compare Hakenberg and Świdrowska, 1998, 2001; Świdrowska and Hakenberg, 1999; Świdrowska, 2007; Świdrowska et a., 2008). The acceptance of this paleotectonic model has some foundational implications: for instance, it was widely assumed that the facies developed within the Mid-Polish Trough would represent the deepest sedimentary environment (e.g., Kutek and Głazek, 1972; Hakenberg and Świdrowska, 1998; Świdrowska 2007). As such, the facies and bathymetric interpretation was evidently fitted to the presumptive paleotectonic model, rather than vice versa. It is noteworthy, however, that Upper Cretaceous mixed carbonate–siliceous facies elude easy environmental interpretation, and the adopted paleobathymetric model and the spatial distribution of particular facies (Fig. 2c) were not supported by any sedimentological features.
Alternatively, another hypothesis has argued for a much earlier onset of inversion tectonics: in this framework, the inversion may have started in the Coniacian and/or Santonian, or perhaps even earlier, in the Late Turonian (e.g., Pożaryski, 1960, 1962; Jaskowiak-Schoeneichowa and Krassowska, 1988; Walaszczyk, 1992; Leszczyński and Dadlez, 1999; Krzywiec et al., 2009; Leszczyński, 2010, 2012; Walaszczyk and Remin, 2015; Remin et al., 2016; Krzywiec et al., 2018; Remin, 2018; Łuszczak et al., 2020).
Based on new sedimentological and chronostratigraphic data, Remin et al. (2015a) and Walaszczyk and Remin (2015) proposed a diametrically opposed sedimentological model (Fig. 2d). Their interpretation suggests the presence of a land area or paleomorphological barrier in the heart of the Mid-Polish Trough, where the deepest part of the basin would have been located according to the model of Kutek and Głazek (1972). Furthermore, their proposed model (Fig. 2d) envisions that the deposits adjacent to the area of epigenetic erosion represent the shallowest facies and pass into deeper facies towards the northeast (Fig. 2d) (Remin et al., 2015a; Walaszczyk and Remin, 2015).
Support of this model (Fig. 2d) is provided by the presence of some specific facies developed locally along the northern edge of the Mid-Polish Anticlinorium: the Turonian Janików Limestone (sandy limestone) characterized by a progradational sedimentary pattern and transport direction towards NE to the flank of the basin (Walaszczyk, 1992) and Campanian–Maastrichtian deltaically or sandy influenced sedimentation in the area of the modern-day Roztocze Hills; both are clearly indicative of a shallow-water environment. It is worth noting that the sandy facies of the Roztocze Hills have been mentioned by several authors (e.g., Kamieński, 1925; Jaskowiak-Schoeneichowa and Krassowska, 1988; Świdrowska et al., 2007; Leszczyński, 2010, 2012; and literature therein), but this prominent terrigenous input has never been explored sedimentologically to reveal their possible paleobathymetry and environment, and source area.
The above interpretation is amply supported by new sedimentological and biotic data (Remin et al., 2015a; Cyglicki and Remin, 2016, 2018; Niechwedowicz et al., 2016; Remin et al., 2016; Remin, 2018). Additionally, extraordinarily preserved plant debris, including complete compound leaves (Halamski, 2013) and characteristic shallow-marine palynofacies (this paper), are strong indicators of proximity to land. In further support of the existence of a paleobiogeographic barrier is its potential impact on paleocirculation, paleotemperature (as derived from oxygen isotopes), the distribution of ammonite fauna (Remin et al., 2016), and the distribution pattern of belemnites during the early Maastrichtian (Remin, 2018).
All those surface observations are in line with seismic data interpretation that shows an overall thinning Upper Cretaceous sedimentary cover towards the SW – i.e., toward the uplifted (at least partly) Mid-Polish Anticlinorium, especially in SE Poland (Krzywiec, 2009; Krzywiec et al., 2009, 2018). Additionally, those data also demonstrate local unconformities (Turonian–Maastrichtian) and progradational bodies directed from the axial part of the basin towards its NE flank (Krzywiec, 2009; Krzywiec et al., 2009, 2018).
It is noteworthy that these two concepts are based more or less on similar input data sets – and in particular, the geometry and thickness patterns of sedimentary successions in cross sections perpendicular to the Mid-Polish Anticlinorium (Fig. 2); however, the processing of those data and final interpretations were different.
Intriguingly, ours is not a novel interpretation. At the beginning of the 20th century, and indeed up until the 1960s (e.g., Rogala, 1909; Nowak, 1907, 1908; Kamieński, 1925; Samsonowicz, 1925; Pożaryski, 1960, 1962), the area of southeast Poland was interpreted as an emerged landmass during the Late Cretaceous. A proliferation of different names were adopted for this landmass, including the “Łysogóry–Dobrogea Land” of Samsonowicz (1925) and Jurkowska et al. (2019a, b); the “Maastrichtian island” (Jurkowska and Barski, 2017); the “Krukienic Island” of Pasternak (1959), Pasternak et al. (1968, 1987), Walaszczyk (1992), and Dubicka et al. (2014); and the “Świętokrzyski Land” or “Małopolska Land” of Pożaryski (1960, 1962) and Jaskowiak-Schoeneichowa and Krassowska (1988), among others.
3.1 The fill of the Polish Basin
The Polish Basin with the Mid-Polish Trough as its most subsiding axial part represented the eastern part of the Central European Basin System (CEBS) formed during the Permian and Mesozoic in the epicontinental basins of western and central Europe (e.g., Pharaoh et al., 2010). In Permian times the Polish Basin constituted the eastern part of the Southern Permian Basin (Kiersnowski et al., 1995; Krzywiec et al., 2017).
From the Permian until the early Late Cretaceous the Polish Basin experienced long-term subsidence (Dadlez et al., 1995) that resulted in its filling of ca. 8000 m of the Permian and Mesozoic deposits, especially along the NW–SE-trending Mid-Polish Trough (e.g., Dadlez et al., 1998; Dadlez, 2003) in the central part of the basin.
During Late Cretaceous time and after the episode of long-term Mesozoic subsidence, the Polish Basin and especially its axial part (the Mid-Polish Trough) underwent uplift and inversion. The inversion tectonics started in the Late Turonian and lasted until the end of the Maastrichtian (or post-Maastrichtian) times (e.g., Krzywiec, 2000, 2002, 2006, 2009; Resak et al., 2008; Krzywiec et al., 2009, 2018).
The Mid-Polish Trough was transformed into a prominent structural unit – the Mid-Polish Anticlinorium (Fig. 1b). At the same time, two marginal troughs were formed: Szczecin–Miechów Synclinorium to the SW and Kościerzyna–Puławy Synclinorium to the NE (Fig. 1b), fed by the elevated areas of the Mid-Polish Anticlinorium. As a result of the inversion process, the Upper Cretaceous deposits are only preserved along both flanks of the Mid-Polish Anticlinorium (Fig. 1b).
The sedimentary cover of the southeastern part of the Polish Basin in SE Poland is different in comparison to its central part where thick Permian, Triassic, Jurassic, and Cretaceous strata accumulated. Namely, only Middle–Upper Jurassic and Cretaceous deposits are well-documented (e.g., Świdrowska et al., 2008 for an overview), whereas the missing part of succession is ascribed to denudation.
In SE Poland (study area), the Middle–Upper Albian (Lower Cretaceous), and the whole Upper Cretaceous succession comprise ca. 1000 m of deposits formed in a rather shallow epicontinental basin (e.g., Jaskowiak-Schoeneich and Krassowska, 1988). Outcrops and well data enabled precise biostratigraphic subdivision based on inoceramids, ammonites, belemnites, echinoids, foraminifera, and to a lesser extent other groups (Walaszczyk et al., 2016). The Upper Cretaceous succession is best exposed in the NE peripheries of the elevated Mid-Polish Anticlinorium where it is accessible in a series of natural and artificial exposures, e.g., in the Middle Vistula Valley Section and the Roztocze Hills area (Figs. 1b and 2a). Starting from the Cenomanian until the Maastrichtian the marine deposits cover most of southeastern Poland and were ascribed to a siliciclastic–carbonate shelf system (e.g., Leszczyński, 1997, 2010, 2012).
3.2 The Roztocze Hills
The Roztocze Hills form a prominent range of hills (approximately 185 km × 25km) that extends from Kraśnik (southeast Poland) to Lviv in western Ukraine along the southwesterly margin of the Kościerzyna–Puławy Synclinorium in southeast Poland (Figs. 1b and 2a). The current-day Roztocze Hills are delineated along the southwest by a prominent escarpment, which constitutes a border zone with the subsurface northeastern edge of the San Anticlinorium underneath the Miocene cover of the present-day Carpathian Foredeep (Figs. 1b and 2a) (e.g., Dziadzio et al., 2006 for further references). In the field, this boundary is manifested as an up to 50–80 m high escarpment, clearly visible from the almost flat surface of the Carpathian Foredeep towards the northeast. The pre-Miocene cover of the San Anticlinorium (southeastern part of the Mid-Polish Anticlinorium) is almost entirely devoid of Mesozoic remnants (with a few exceptions; Fig. 2a) and is represented mainly by Cambrian and Neoproterozoic, strongly tectonized and weakly metamorphosed (anchimetamorphic) flysch-type deposits of an unknown thickness (e.g., Dziadzio and Jachowicz, 1996; Buła et al., 2008; Żelaźniewicz et al., 2009; Buła and Habryń, 2011).
This prominent tectonic line has been variously interpreted by different workers (e.g., Kutek and Głazek, 1972; Narkiewicz et al., 2015; Narkiewicz and Petecki, 2017, for reviews). It is now considered that the southwestern edge of the Roztocze Hills coincides with crustally rooted fault zones marked as nearly vertical crustal-scale conductive zones (review in Narkiewicz et al., 2015; Narkiewicz and Petecki, 2017), that were repeatedly reactivated during the Late Cretaceous inversion of the Mid-Polish Trough as a reverse fault (Krzywiec, 1999; Krzywiec et al., 2009) and by Carpathian tectonics during the Miocene (Kowalska et al., 2000; Buła et al., 2008; Buła and Habryń, 2011).
In the Polish part of the Roztocze Hills, the range is made up of Campanian (up to 550 m thick) and Maastrichtian (ca. 250 m or more, but incomplete) deposits; older deposits of the Lower–Upper Cretaceous succession are not exposed. From place to place the Upper Cretaceous is unconformably overlain by Miocene sediments (e.g., Pożaryski, 1956). Depending on the section, the Upper Cretaceous deposits of the Roztocze Hills are represented by various opoka facies (siliceous chalk or limestone with a variable admixture of biogenic silica), gaizes (siliceous limestone with a considerable admixture of detrital quartz, glaucony, and clay), marls, calcareous sandstones, and calcareous mudstones, in addition to argillaceous mudstone or clays, the latter sometimes devoid of any CaCO3 admixture. Regionally, the succession dips gently to the northeast.
3.3 The Szozdy section
The Szozdy section is situated within a railroad cut of the Broad Gauge Metallurgical Railway Line, about 4 km southwest of Zwierzyniec close to the small village of Szozdy in southeast Poland (Fig. 3). The studied interval has yielded a rich fossil assemblage, comprising ammonites, belemnites, inoceramid, non-inoceramid bivalves, echinoids, diverse gastropods, and a suite of microfossils (Remin et al., 2015a, b) in addition to partially carbonized plant debris such as leaves or boughs (Fig. 3).
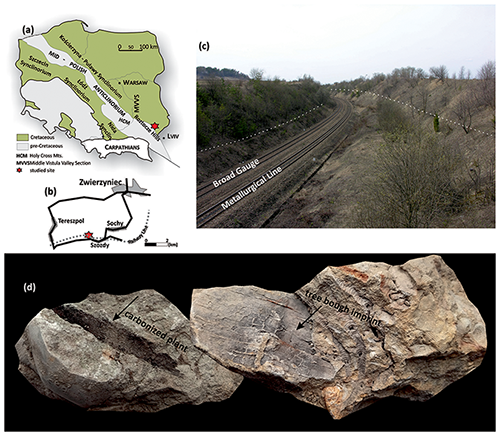
Figure 3(a) Simplified geological sketch map of extra-Carpathian Poland, excluding the Cenozoic cover (adopted from Pożaryski, 1974); tectonic units after Żelaźniewicz et al. (2011). (b) Detailed location of the studied section. (c) General view of the section – the exposures (white dashed lines) extend along both sides of the railroad cut over a distance of approximately 800 m. (d) Carbonized plant fragment (left) – the specimen is ca. 10 cm across; the imprint of tree bough (right) – the specimen is ca. 25 cm across.
This fossil assemblage defines a middle Campanian age for the Szozdy section (Remin et al., 2015a): specifically, the lower–middle Didymoceras donezianum ammonite zone (sensu Błaszkiewicz, 1980) and the middle “Inoceramus” tenuilineatus inoceramid zone (Walaszczyk, 2004) (Fig. 4). The interval available at Szozdy, together with other sections in the Roztocze Hills, is easily correlatable with the equivalent interval in the Middle Vistula River Valley section (Fig. 4; for location compare Figs. 1b and 2a), a reference section for the Upper Cretaceous of the southeastern Polish Basin (e.g., Walaszczyk, 2004; Walaszczyk et al., 2016).
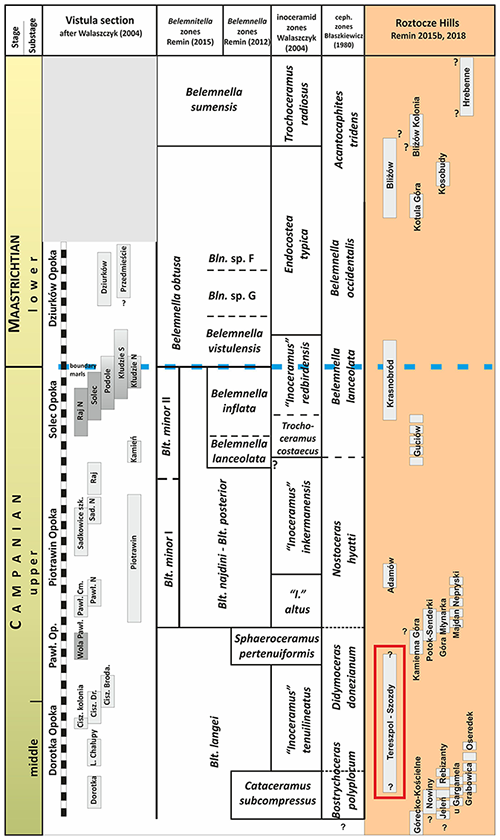
Figure 4The stratigraphic position of the Szozdy section (right column; scale not included relative to the Middle Vistula River composite section (left column; scale bar = 2 m). A full description of the other sections of the Roztocze Hills and their stratigraphic position will be provided elsewhere. The biostratigraphic subdivision follows Błaszkiewicz (1980), Walaszczyk (2004), Remin (2012, 2015), Remin et al. (2015b), and Remin (2018).
3.4 Material and methods
The Szozdy section was sampled for (1) sedimentological evaluation, including both macro- and micro-scale observations, (2) heavy minerals, and (3) palynofacies analysis, the latter two mainly to examine hydrodynamic properties and paleoenvironmental context, respectively.
Of 22 total samples, 17 (1–17) were subjected to detailed analyses: all of these were collected from the lower portion of the section (Fig. 5). This part of the section (cycles II–IV; Fig. 5), as the most densely sampled, was chosen to examine the differences and/or regularities governing the distribution of heavy minerals (Fig. 6) and palynofacies indicators, complementing sedimentological observations.
In the present study, the Szozdy section is placed within the wider context of CaCO3, quartz sand, clay content, plant debris, and thickness distribution patterns for the Campanian strata of the Middle and Eastern Roztocze Hills (Fig. 7).
The thickness pattern of the Campanian deposits in the Roztocze Hills area used in this study is based on 16 boreholes with documented Campanian deposits: Chrzanów IG-1, Dyle IG-1, Izbica IG-1, Jarczów IG-2, Jarczów IG-4, Komarów IG-1 – IG4, Narol PIG-1, Narol PIG-2, Rachanie IG-1, Stróża IG-1, Tarnawatka IG-1, Tomaszów Lubelski IG-1, and Ulhówek IG-1 (Fig. 8). The well-log data are stored in the Central Geological Database (CBDG; http://baza.pgi.gov.pl/, last access: 3 January 2022) of the Polish Geological Institute – Polish Research Institute (PIG-PIB). The stratigraphic data, especially the lower and upper limit of the Campanian stage (where available), are based on data stored in the CBDG database for particular boreholes. Where necessary, well-log data were interpolated using the kriging and TopoToRaster geostatistic tools of ArcGIS.
Sedimentology. The scope of sedimentological analysis included both macro- and microscale (i.e., thin sections) observations. During fieldwork, the main lithofacies and thicknesses of the successive units were recorded. Persistent sedimentary structures – i.e., a coarsening upward succession within the recognized cycles, trace fossils (bioturbation), macrofossil content, and micro-bioturbation (the latter within thin sections) – were also noted.
CaCO3 contents were measured in the Polish Geological Institute (PIG-PIB) at over 50 localities (and ca. 3 times more measurements). Thin section analysis enabled a volumetric estimation of the main granular components, including quartz, glauconite, and biogenic calcium carbonate. Silt and clay content were roughly estimated based on the volume of the granular and CaCO3 components.
Heavy mineral analysis. In the present study, the heavy mineral analysis includes both qualitative and quantitative analyses of heavy mineral assemblages (here, operationally defined as having a density > 2.9 g cm−3). A combination of optical microscopy, scanning-electron-microscope energy-dispersive X-ray spectroscopy (SEM-EDS), and electron microprobe (EPMA) analyses were applied (Mange and Maurer, 1992; Velbel, 1999, 2007; Velbel et al., 1996, 2007; Turner and Morton, 2007; Andò et al., 2012; Garzanti and Andò, 2019). EPMA analyses included an examination of tourmaline and garnet geochemistry (e.g., Wright, 1938; Henry and Guidotti, 1985; Preston et al., 2002; Mange and Morton, 2007; Meres, 2008; Andò et al., 2013).
Palynofacies analysis. Following Tyson (1993, 1995), kerogen particles were subdivided into phytoclasts (opaque phytoclasts, translucent phytoclasts, cuticle), palynomorphs (terrestrial, marine), and amorphous organic matter (AOM). A minimum of 300 kerogen particles per sample were counted for palynofacies analysis. A preliminary analysis of palynomorph assemblages is focused on both sporomorphs (spores, pollen, saccate pollen) and aquatic palynomorphs (dinoflagellate cysts, foraminiferal test linings, acritarchs, algae, and freshwater algae), based on at least 250 counts per sample. Additionally, the terrestrial to marine (T M) palynomorph index and peridinioid to gonyaulacoid (P G) dinoflagellate cyst ratio (see, for example, Olde et al., 2015) were also ascertained.
4.1 Sedimentology – macro-and microscopic observations in the Szozdy section
The most prominent feature of the Szozdy section is the presence of tripartite cyclothems. Where complete, an individual cyclothem consists of three units: from bottom to top, calcareous mudstone, calcareous sandstone, and calcareous gaize (Fig. 5). Not every cyclothem is complete within the study section: for instance, cyclothem II lacks the calcareous sandstone unit (Fig. 5).
Presently, we cannot estimate the total thickness of the Szozdy deposits represented by tripartite cyclothems, since neither the lower nor upper limit of the cyclic succession is known. The exposure at Szozdy (Fig. 3) gives access to approximately 30–35 m of the succession over a distance of ca. 800 m. Other natural or artificial exposures are not present; furthermore, well-log data are not available in close proximity to the Szozdy section. In the more distal boreholes, the Late Cretaceous rocks were obtained as cuttings only: therefore, the existing well-log data preclude any firm conclusions. For the present study, we choose a 12 m thick interval that consists of six cycles including both complete and incomplete ones (Fig. 5).
The calcareous mudstone lithofacies (Fig. 5) is dark grey and poorly indurated. The color is most likely derived from disseminated carbonized organic matter. Of the distinguished lithofacies, the clay and silt content is highest in the calcareous mudstone and ranges from 31 %–44 % (mean: 38 %). The sand fraction is composed mainly of quartz and ranges from 16 %–26 % (mean: 22 %): it is the lowest within the whole section. The quartz is fine-grained, with a mean value of ca. 0.1 mm; the grains are sub-angular to angular, with, less commonly, sub-rounded grains. In successive cyclothems, the CaCO3 content of this unit varies over a relatively narrow interval (ca. 34 %–46 %; mean: 38 %) and is ascribed mainly to broken biocomponents. In outcrops, small bioturbation structures are visible: other sedimentary structures are absent. In this unit, macrofauna is rare: only some bivalves and badly preserved echinoids were found.
The calcareous sandstone lithofacies (Fig. 5) is yellow to yellow-brownish and poorly indurated. The clay and silt content is ca. 10 % lower than in the underlying calcareous mudstone, ranging from 24 %–36 % (mean: 29 %). The sand fraction, largely consisting of quartz and, to a subordinate extent, glauconite, varies in a narrow interval (36 %–43 %; mean: 40 %): these values are the highest of the lithofacies recognized in the Szozdy section. The quartz sand is still fine-grained: however, the size of the grains is up to approximately 0.2 mm, twice as large relative to the underlying calcareous mudstones. The quartz grains are sub-angular to angular – sub-rounded grains are somewhat less common. The CaCO3 content is ca. 10 % lower relative to calcareous mudstone, ranging from 26 %–38 % (mean: 31 %). In outcrops, this unit seems to be bioturbated at least to some degree; correspondingly, sedimentary structures are absent. Macrofauna is extremely rare in this lithofacies: similarly to the underlying calcareous mudstone, only some badly preserved bivalves were found.
The calcareous gaize lithofacies (Fig. 5) is white-gray, fully indurated, and can be extremely hard; in the field, it is expressed as protruding horizons between more erosion-prone deposits (Fig. 5). This unit is composed of a limited clay and silt fraction, ranging from 8 %–18 % of the bulk rock (mean: 13 %): these are the lowest clay and silt fractions of the lithofacies recognized in the Szozdy section. The sand fraction, consisting of quartz sand with, to a subordinate extent, glauconite, ranges from 21 %–35 % (mean: 24 %): the sand fraction is similar to that recognized in the underlying unit but is fully cemented by a calcareous cement, with only a subordinate siliceous cement. The CaCO3 content is the highest out of the recognized lithofacies: 60 %–75 % (mean: 64 %). Of the three lithofacies of the full cyclothem, only this lithofacies yielded well preserved marine fauna, consisting of relatively frequent ammonites (including baculites), inoceramids, and, extremely rarely, belemnites and solitary corals. Other faunas include non-inoceramid bivalves and different snail species. It is worth mentioning that ammonites and other aragonitic shell material frequently retain remnants of the original shell structure, including iridescence. This unit also yielded abundant macroscopic plant debris, including tree boughs, tree branches, and relatively well-preserved compound leaves and/or their fragments.
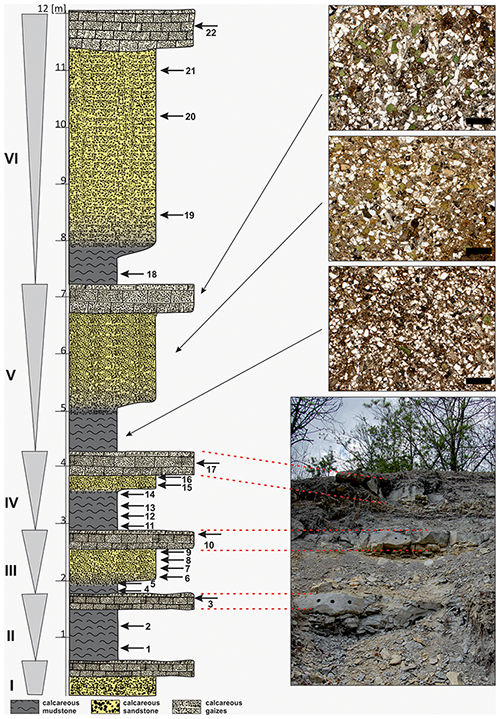
Figure 5Lithological column of the Szozdy section. Triangles on the left side of the column indicate coarsening-upward cyclothems. Top right: typical thin sections from successive lithofacies of a single cyclothem; scale bar = 500 µm; most of the white particles represent quartz grains. Bottom right: field photo of a part of the secession with prominent layers of calcareous gaize, indicated on the section by red dashed lines. The horizontal scale represents relative resistance to weathering of particular units.
4.2 Heavy minerals
The heavy-mineral assemblages from the mixed calcareous siliciclastic deposits of the Szozdy section are characterized by a stable composition, with little differentiation. The analyzed associations included ultrastable and stable phases: in order of decreasing resistance to weathering under surface conditions, these include rutile, zircon, tourmaline, sillimanite, kyanite, staurolite, garnet, authigenic glauconite, pyrite, and other subordinate species such as chlorite, Cr-spinel, epidote, and single apatite grains.
The zircon–tourmaline–rutile (ZTR) maturity index (Hubert, 1962) ranges between 55 %–85 % (mean: 67 %). The highest ZTR values are derived from the calcareous mudstone lithofacies and driven mainly by the tourmaline abundance (dravite to shorl ratio, 4:1). In general, of the three ZTR index mineral species, tourmaline dominated in all of the studied samples. Amongst the ZTR minerals, subrounded and rounded crystals dominate (> 65 %); to a much lesser extent, other ZTR crystals are angular or even euhedral (ca. 4 %). On the other hand, garnet, kyanite, and staurolite crystals are almost exclusively angular (> 70 %–80 %), with almost no oval and/or rounded ones.
In the lower part of the Szozdy section (which, as described above, was chosen for detailed studies), a distinct rutile and tourmaline abundance pattern can be observed – namely, that these two mineral phases are inversely correlated (Fig. 6). It is worth noting that the grains of both mineral phases are of similar size of ca. 100–140 µm in each unit. This is best observed in cyclothems III and IV (Fig. 6), which are the most densely sampled. In the calcareous mudstone unit of cyclothem III, the abundance of rutile is markedly reduced, whereas the tourmaline content is significantly higher than average. The opposite pattern is observed in the calcareous sandstone overlying the dark mudstone: here, rutile abundance is substantially elevated, while the tourmaline content is lower than average. A similar pattern to in the sandstone lithofacies is observed within the calcareous gaize lithofacies (Fig. 6). The only exception to this general trend is the calcareous gaize unit at the top of the (incomplete) cyclothem II, in which the sandstone lithofacies is absent. In this case, the rutile–tourmaline abundance trends follow the pattern recognized in the underlying mudstone.
To emphasize the relative changes in abundance of these two mineral species, a standardized Z-score statistic was calculated (Fig. 6). This statistic quantifies how many standard deviations each sample is from the mean value for the whole group for the entire Szozdy section (for comparison: Ryan et al., 2007).
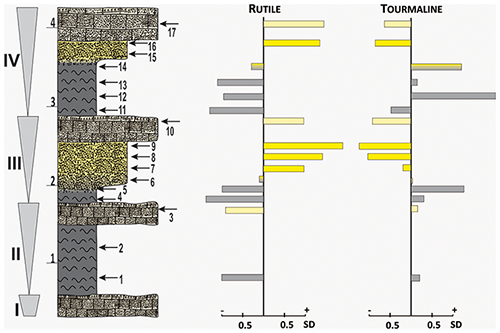
Figure 6Vertical chart of Z-score standardized rutile and tourmaline abundance in the Szozdy section. The vertical line represents the mean abundance of the analyzed minerals over the whole section. The bars are associated with the equivalent samples shown on the stratigraphic column; SD – standard deviation.
4.3 Palynofacies
Terrestrially sourced organic matter, represented by the phytoclast group (opaque phytoclasts, translucent phytoclasts, and cuticle), is the dominant palynofacies component throughout the studied succession. The AOM content is generally low. Among the terrestrially derived palynomorphs, sporomorphs (spores, pollen, saccate pollen) are well-represented, while freshwater algae are rare. Marine palynomorphs are represented by dinoflagellate cysts, foraminiferal test linings, acritarchs, and algae. Dinoflagellate cysts and foraminiferal linings are the most common marine palynomorphs, although their abundances fluctuate throughout the succession; acritarchs and algae are less common but have relatively consistent abundances. The dinoflagellate cyst assemblages are rich and diverse; gonyaulacoid dinoflagellate cysts are generally more common than peridinioids.
Calcareous mudstone. In this lithofacies, palynofacies are dominated by phytoclasts (ca. 85 %–93 % of total kerogen), of which translucent phytoclasts (ca. 33 %–44 % of total kerogen) and cuticle (ca. 22 %–42 % of total kerogen) are the main constituents. The concentration of cuticle in calcareous mudstones is visibly higher than in other lithofacies; however, the cuticle debris is largely degraded. The content of AOM fluctuates, but at low concentrations (0.4 %–5 % of total kerogen). The terrestrial marine ratio is relatively high, ranging between 26 % and 41 %, and is ascribed mainly to the abundance of non-saccate pollen (up to 82 % of sporomorphs). Foraminiferal linings (ca. 44 %–64 %) are clearly more abundant than the other marine palynomorphs. The P G ratio is variable (11 %–39 %) and indicates that peridinioid dinoflagellate cysts are comparatively less common in calcareous mudstones relative to calcareous sandstones.
Calcareous sandstone. This lithofacies is characterized by the dominance of phytoclasts (up to 79 % of total kerogen), which are mainly represented by translucent phytoclasts (ca. 43 %–63 % of total kerogen). Of note are the very low concentrations of cuticle, which constitute only up to 3 % of the total kerogen. The AOM content is generally low (ca. 0.5 %–2 % of total kerogen) but may occasionally reach higher values (ca. 15 %). Calcareous sandstones are characterized by the highest T M ratios (up to 49 %) in the entire section; elevated saccate pollen concentrations (up to 51 % of sporomorphs) are notable. Marine palynomorphs are dominated by dinoflagellate cysts; peridinioids are visibly more common (P G = 28–45 %) in calcareous sandstones compared to other lithofacies. Conspicuously, foraminiferal linings are almost absent.
Calcareous gaize. Phytoclast concentrations, although still relatively high (ca. 44 %–67 % of total kerogen) and dominated by translucent phytoclasts (ca. 18 %–44 % of total kerogen), are visibly lower compared to the other lithofacies. Cuticle abundance is low to moderate (6 %–22 % of total kerogen), and the cuticle debris is mostly degraded. The AOM content is generally high (ca. 35 %–47 % of total kerogen): however, occasionally it may be somewhat lower (ca. 7 %). The T M ratio (27 %–33 %) is visibly lower compared to other distinguished lithofacies, with non-saccate pollen dominant over sporomorphs. Dinoflagellate cysts predominate over the other marine palynomorphs, and the P G ratio exhibits moderate values (19 %–32 %).
4.4 Distribution of CaCO3 and thickness patterns in Campanian deposits
To place the middle Campanian succession of the Szozdy section in a wider sedimentological context, two maps were constructed. The first (Fig. 7) shows the distribution pattern of CaCO3 content coupled to terrigenous material (quartz sand and clay) content and is based on more than 50 discrete outcrop observations (green triangles in Fig. 7) and ca. 3 times as many measurements. In most of the outcrops, two types of lithologies were available – less and more clayly. The map is constructed based on the lowest available measurements from particular outcrops. CaCO3 content is more or less inversely correlated to quartz sand and clay content. Additionally, the distribution pattern of macroscopic plant debris is provided (Fig. 7), including imprints of tree boughs, tree branches, and leaves. The frequency of plant debris is described by two subjective classes – rare and common – based on field observations in particular outcrops (Fig. 7).
The lowest CaCO3 values are concentrated in a relatively narrow belt, bordered to the southwest by the present Carpathian Foredeep (the subsurface northeastern edge of the San Anticlinorium) (Figs. 2a and 7): correspondingly, this is the area with the highest abundance of terrigenous material (quartz sand and clay) and plant debris. Along a SW–NE transect, the CaCO3 content progressively increases, whereas the quartz sand and clay content tend to decline towards the northeast. It is worth observing that in the southeast part of the studied area – i.e., the Horyniec Zdrój area (Fig. 7) – pure clay intervals may also occur in couplets with clayey opokas.
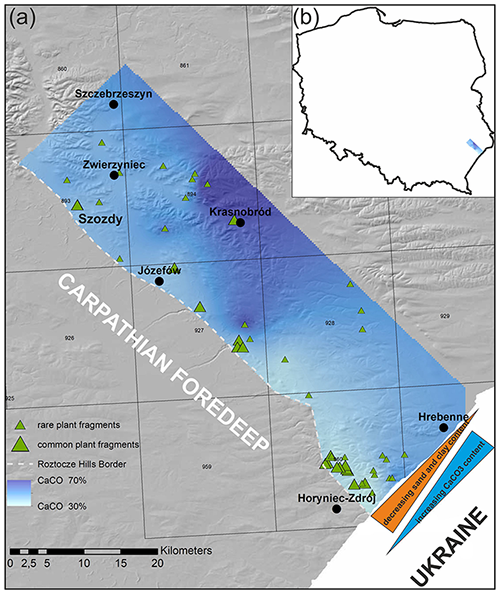
Figure 7(a) Distribution of CaCO3 in the Roztocze Hills area between Zwierzyniec and the Polish–Ukrainian border to the east. Note that CaCO3 is consistently lowest at the edge of the Roztocze Hills, at the geologic border with the Carpathian Foredeep. Green triangles indicate findings of macroscopic plant fragments; (b) general map of Poland with study area. Squares with numbers represent the associated geological map sheets (https://geologia.pgi.gov.pl/, last access: 3 January 2022).
The second map shows the thickness pattern of Campanian deposits in the Roztocze Hills, based on 16 boreholes with appropriate interpolations (see methods for further details). It is worth mentioning that the original thicknesses, especially in the most southwesterly part which borders the Carpathian Foredeep (subsurface northeastern edge of the San Anticlinorium – compare Figs. 2a and 8) may be even higher since the entire Campanian is not preserved locally. The highest values are located in the vicinity of the Narol boreholes (Fig. 8), where the Campanian reaches ca. 550 m and along the southeastern edge of the Roztocze Hills. To the northeast, the thickness of the Campanian deposits progressively decreases, declining to ca. 150–200 m in the Komarów and Ulhówek boreholes (Fig. 8).
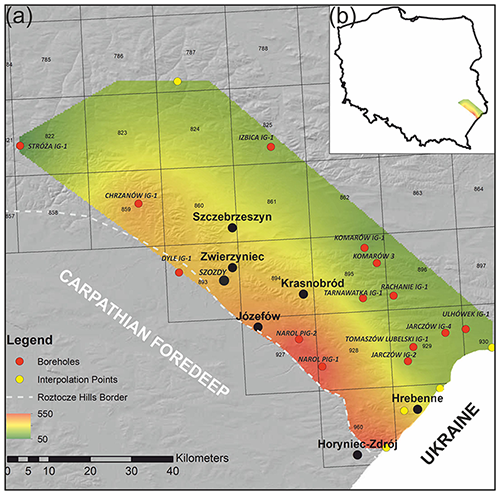
Figure 8(a) Thickness pattern of Campanian deposits in the Roztocze Hills based on well data; (b) general map of Poland with study area. Squares with numbers represent the associated geological map sheets (https://geologia.pgi.gov.pl/, last access: 3 January 2022).
The studied section is special: similar lithologies have not been described previously either from the Roztocze Hills area or from any other part of the Polish Basin. Even the boreholes from the vicinity of the study section did not provide a similar sedimentological pattern. The Szozdy section is characterized by coarsening-upward tripartite cyclothems represented by mixed carbonate–siliciclastic sediment rich in terrestrial components. The presence of frequent plant debris, i.e., imprints, carbonized fragments and disseminated organic matter of phytogenic origin, and scarcity of macroscopic, fully marine fauna in selected units of the cyclothems (the calcareous sandstone) point to its origin in close proximity to land areas and at least some interplay of marine and fresh waters (compare below). For those reasons, Remin et al. (2015a) suggested a deltaically influenced sedimentation in the Szozdy area. Such an interpretation and especially shallow, marginal marine sedimentary environments (Fig. 2d) located close to the elevated Mid-Polish Anticlinorium were recently independently confirmed by Jurkowska and Barski (2017) based on palynofacies analysis, Jurkowska et al. (2019a, b) based on overall diagenetic patterns, and Machalski and Malchyk (2019) based on distribution and taphonomy of macrofauna.
Additionally, the prominent quartz sand input and terrestrially rich components and their progressive disappearance towards the NE (Fig. 7), thus outside the uplifted Mid-Polish Anticlinorium, also suggest the proximity of land which is considered to have been located over the present-day San Anticlinorium. Although the sand-rich facies in the Roztocze Hills has been mentioned by several authors (e.g., Kamieński, 1925; Pożaryski, 1956, 1960, 1962; Jaskowiak-Schoeneichowa and Krassowska, 1988; Hakenberg and Świdrowska, 2001; Świdrowska, 2007; Świdrowska et al., 2008; Leszczyński, 2010, 2012), these quartz- or clay-rich deposits have never been explored sedimentologically, to place them within a paleobathymetric or paleoenvironmental and sedimentological context.
Although similar cyclic sedimentation can be recognized in several marginal marine environments (lower shoreface, storm deposits, tide-dominated deltas, and more offshore shelf deposits) we retain the opinion that the Szozdy section is most easily evaluated if a deltaic origin of those tripartite cyclothems is accepted. The upper and lower shoreface together with storm deposits, if any, will produce cyclicity with normal grading, thus opposite to the Szozdy case; tide-dominated deltas are mainly characterized by millimeter- to centimeter-scale rhythmites (Kvale, 2012); whereas the more offshore settings might be precluded since there is no chance for the influence of freshwater suggested by the palynofacies analyses (compare below).
Besides macro- and microscopic sedimentological characteristics, the deltaic origin of the Szozdy section is here independently supported by the palynofacies analysis and hydrodynamic properties derived from heavy mineral analysis (as elaborated below) in addition to the distribution patterns of siliciclastic material and CaCO3 in a wider context (Fig. 7).
5.1 Palynofacies analysis
The characteristic palynofacies features of the Szozdy succession include abundant phytoclasts (particularly translucent phytoclasts and cuticle), sporomorphs, peridinioid dinoflagellate cysts, and foraminiferal linings, all of which are indicative of a relatively proximal depositional setting. In particular, the abundance of translucent phytoclasts – that is, fresh, unoxidized particles – are suggestive of very short and rapid transportation (Tyson, 1993). A similar inference may be made from the abundance of cuticle particles, which can easily be degraded and, thus, may serve as an indicator of rapid transportation (e.g., Tyson, 1993). The relatively high concentrations of sporomorphs, and particularly the presence of spores and non-saccate pollen, support this interpretation, as these sporomorphs are preferentially deposited in close proximity to land (Tyson, 1993). The elevated percentages of peridinioid dinoflagellate cysts and foraminiferal linings are likely related to increased availability of nutrients, which may either originate from upwelling (e.g., Wall et al., 1977; Lewis et al., 1990; Powell et al., 1990, 1992; Eshet et al., 1994), or riverine discharge (e.g., Downie et al., 1971; Wall et al., 1977; Powell et al., 1990, 1992; Hardy and Wrenn, 2009). In the present case, the latter source of nutrients seems more probable, judging from the composition of the phytoplankton assemblage (e.g., the absence of typically oceanic dinoflagellate cyst genera, such as Impagidinium and Nematosphaeropsis, and the presence of estuarine gymnodinioid dinocysts).
The cyclic fluctuations in the palynofacies and palynomorph assemblages characteristic of particular lithofacies suggest highly dynamic conditions. The most evident differences exist between the calcareous mudstones and sandstones (Fig. 9), as revealed by the changing relative percentages of phytoclasts (mainly cuticle), pollen, peridinioid dinoflagellate cysts, and foraminiferal linings. Such a sharp transition between the palynofacies patterns could be explained by either varying salinity levels, the different hydrodynamic properties of palynofacies components, or a mixture of both. Both dinoflagellate cysts and foraminiferal linings are indicative of relatively normal salinity conditions, although some dinoflagellates might have been tolerant of abnormal salinities in nearshore settings (Tyson, 1993). Hence, the rarity of foraminiferal linings in the calcareous sandstone units could possibly be explained by the influence of brackish conditions. The almost complete absence of cuticles in calcareous sandstones (Fig. 9) may also indicate higher flow conditions, resulting in the continued suspension of the most buoyant particles, such as cuticle (see Tyson, 1993), and subsequent bypassing via floatation and deposition in more distal, lower-energy settings (e.g., calcareous mudstones and gaizes) (Fig. 10). On the other hand, the elevated percentages of saccate pollen in calcareous sandstones may suggest the opposite – a decrease in water energy, since saccate pollen are preferentially concentrated in low-energy environments (see Tyson, 1995). Nevertheless, the slightly higher T M and P G ratios recorded in calcareous sandstones (Fig. 9) suggest that these lithofacies were deposited more proximally than the calcareous mudstones and gaizes (Figs. 9 and 10).
The palynofacies patterns and palynomorph assemblages documented from calcareous mudstones and gaizes (Fig. 9) are less spectacular. In both lithofacies, the phytoclast content (including the cuticle) is comparable and relatively high. Dinoflagellate cysts and foraminiferal linings are also well represented in both units, although the proportion of the latter is visibly lower in calcareous gaizes. Gaizes are also characterized by elevated AOM concentrations (the highest values recorded throughout the succession), suggestive of a more distal setting for this lithofacies (see Tyson, 1993, 1995). Considering the above, it is reasonable to suggest that calcareous sandstones represent the most proximal setting, while gaizes were apparently deposited in a more offshore environment (or eventually experienced less material supply); mudstones occupied an intermediate position (compare Fig. 10).
5.2 Hydrodynamic properties of the environment
The strong inverse relationship between tourmaline and rutile can be readily recognized throughout the whole section (Figs. 6 and 9), indicating systematic changes in hydrodynamic power in the sedimentary environment.
The Z-score calculations, normalized to the whole study section, underline the changes in the proportion of respective minerals in succeeding samples, enabling a better understanding of the processes governing their distribution pattern within the section (Fig. 6) (e.g., Ryan et al., 2007). Both minerals are of similar shape, durability, and size: however, they possess markedly different densities – namely, 3.03–3.18 and 4.23 g cm−3 for tourmaline (dravite) and rutile, respectively. Since the weight of the two analyzed minerals is high, it might be expected that these two mineral phases will be strongly dependent, both vertically and spatially, on the local sedimentary environment – and by extension, the hydrodynamic power that existed during the deposition of the cyclothem. The recurring increase in tourmaline abundance, coupled with a simultaneous decrease in rutile abundance in muddy units, most likely resulted from a decrease in hydrodynamic power in the depositional environment. Sedimentologically, this may be interpreted as a transition to a comparatively more distal depositional setting from the main source area, e.g., a river discharge – that is, the prodelta or analogous environment (compare Figs. 9 and 10) (Komar, 2007; Omran, 2007).
Analogously, the increase in the proportion of rutile, and the simultaneous decrease in tourmaline share, in the sandy units can be linked to an increased flow rate, which might be interpreted as a transition to an environment more proximal to the river discharge, thus representing the main delta lobe and/or slope setting (compare Figs. 9 and 10). Simply put, the (lighter) tourmaline will be transported further to the prodelta environment, rendering the prodelta facies overrepresented in this mineral phase, whereas the (markedly heavier) rutile will be deposited closer to the river discharge.
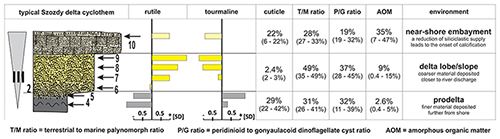
Figure 9The proposed environment for the successive lithofacies within an individual cyclothem in the Szozdy delta, coupled with the vertical distribution of selected heavy minerals and palynofacies indicators: cyclothem III adopted from Fig. 6. The values in brackets show the range for the lithofacies across all studied cyclothems. SD: standard deviation.
5.3 Sedimentary environment – looking for recent counterpart
We found that the modern Mahakam deltaic system (Indonesia) may serve as a good modern counterpart for the Szozdy Delta sedimentary environment. The similarities are, of course, general – the Mahakam Delta is huge in comparison to the Szozdy deltaic system (although the spatial and vertical distribution of the latter is unknown); however, beyond those differences, several analogies can be found in their respective sedimentary environments.
The presence and relative importance of physical processes (e.g., water flow, sediment input, ocean currents, wind, waves, and tides) regulate the morphology and internal geometry of deltas. Accordingly, three main classes are commonly distinguished: (i) tide-dominated, (ii) wave-dominated, and (iii) river-dominated deltas (e.g., Coleman and Wright, 1975; Galloway, 1975).
The modern Mahakam delta is considered a textbook example of a fluvial-tide-dominated delta system built across a narrow shelf (Galloway, 1975; Allen et al., 1977). The shelf and deltaic deposits border the N–S-oriented Makassar Trough, which acts as a throughflow pathway for Pacific waters to the Indian Ocean (Wyrtki, 1987); as such, it must also regulate, at least in part, the redistribution of suspended sediments. The Mahakam River discharge is characterized by a distinct absence of flood surges: therefore, the avulsion of distributary channels does not typically occur (e.g., Allen et al., 1977; Storms et al., 2005). Additionally, the extremely wide, submerged delta front platform, which extends as a 5 m isobath up to 15–20 km offshore, works as an ideal platform to dissipate wave energy (Roberts and Sydow, 2003). As a consequence, the fluvial distributaries efficiently push suspended sediment basinward, resulting in extremely fast progradation (Storms et al., 2005, and references therein).
For the Szozdy Delta, it is difficult to ascertain which process(es) were dominant in the development of this deltaic system (fluvial-, tide-, and/or wave-dominated): at a minimum, such assessments are speculative to at least some degree. Although relatively large, the exposure at Szozdy – an 800 m long railroad cutting that provides access to approximately 30–35 vertical meters of the succession still represents a single locality, with such a specific sedimentation style that can be best described as deltaic in origin.
On the other hand, it is worth accounting for the fact that successive cyclothems are very regular, and particular units can be traced over dozens to a few hundred meters. Strong wave and/or tidal action would most likely result in a more chaotic development of the successive lithofacies, and ultimately entire cyclothems. Accordingly, at the current stage, we can only speculate that fluvially dominated processes are predominant, with possibly some additional wave and/or tidal action.
The limited thickness of the recurring cyclothems – 1–5 m (Fig. 5) – and upwards grain-size coarsening patterns in each cyclothem indicate that the progradation of succeeding facies – i.e., the transition between the more muddy and more sandy lithofacies of the cyclothems (Figs. 5 and 9) – proceeded relatively quickly. This also suggests that accommodation space was highly limited (Fig. 10).
Since there is no evidence for the presence of delta plain deposits, it is suggested that the entirety of sedimentation occurred in the subaquatic part of the Szozdy deltaic system, as exemplified in Fig. 10.
The implication is that, at least locally, the deposition of the submerged deltaic facies of the Szozdy delta took place along a relatively flat, wide, shallow to extremely shallow delta front platform (Figs. 10 and 11), similar to the delta front platform of the modern Mahakam delta, where progradation is fastest. The platform was wide enough to dissipate most of the wave-energy action, rendering the influence of waves low to moderate, at a maximum.
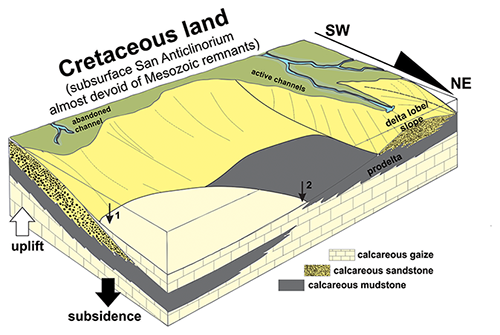
Figure 10Block diagram illustrating the relationships between the subaerial (not available in the study area) and subaqueous deltaic environments of the Szozdy delta in relation to active and abundant distributary channels. Arrows 1 and 2 indicate two different situations, i.e., (1) a full tripartite cyclothem and (2) an incomplete cyclothem; compare to Fig. 5. Not to scale.
The differing thickness of successive lithofacies in the cyclothems (Fig. 5) suggests that specific environmental conditions had different durations before a threshold was reached that drove a change in paleoenvironmental regime from prodelta to delta lobe/slope facies. This suggests that rather dynamic tectonic conditions existed, resulting in changes of the delta architecture, the migration and avulsion of distributary channels, and simultaneous changes in the sedimentary environment at particular localities, resulting in the repetition of cyclothems.
According to this proposed environmental interpretation, the lowest unit of each cyclothem – that is, the calcareous mudstone – would represent the prodelta environment (Figs. 10 and 11), deposited on the submerged delta front platform in a comparatively distal region from the river discharge (Figs. 10 and 11). This unit possesses the highest clay abundances and the finest sand fraction. Upsection, the calcareous mudstone passes quickly into yellowish calcareous sandstone, with markedly less disseminated organic matter of phytogenic origin. In additional support of such an environmental interpretation is the scarcity of macroscopic, fully marine fauna: while some bivalves and echinoids were recognized in the mudstone lithofacies, in the sandstone lithofacies such fauna is almost absent.
The origin of the calcareous gaize is more problematic. This lithofacies is considered to originate in an area cut off from the sediment supply. Once the siliciclastic sediment supply is inhibited – for instance, via tectonic activity and/or changes in delta architecture (e.g., the avulsion of distributary channels) – a relatively quick calcification process is thought to quickly lithify and “freeze” the upper portion of the sediment.
Three observations support such an interpretation. First, in all studied cases, the dominant grain size is always the same as the grain size observed in the underlying unit. In complete cyclothems (i.e., cyclothems I and III–VI), the gaize possesses a grain size similar to the sandy unit (Fig. 5). In cyclothem II, where gaize directly overlies the muddy unit, the grain size is smaller and follows that observed in the mudstone. Second, in this unit, fully marine fauna are quite common, which indicates that the freshwater supply was comparatively limited or redirected. Additionally, terrestrial components are also abundant. In particular, macroscopic plant debris, including tree boughs, tree branches, and compound leaves, are commonly found: it is suggested that buoyant fragments were transported, sunk, and subsequently “frozen” by calcification. Third, ammonites and other aragonitic shelled animals still retain remnants of the original shell structure, including their iridescence. This indicates that they were cut off from the surrounding environment, which would have assuredly been corrosive to aragonite – in other words, the original aragonite could not have been preserved without syndepositional and/or early diagenetic calcification and chemical stabilization.
5.4 Towards an understanding of Late Cretaceous facies distribution
The very existence of a middle Campanian deltaic system developed in the axial part of the inverting Mid-Polish Trough (into the Mid-Polish Anticlinorium) has some fundamental consequences for our overall understanding of certain Late Cretaceous facies. In particular, this concerns mainly opoka, chalk, and their respective variants, in addition to their mutual spatial and bathymetric relationships in transects perpendicular to the Łysogóry–Dobrogea Land (Fig. 2d).
The evidence presented here for shallow-water, deltaic origin of these mixed carbonate siliciclastic deposits confirms the conceptual proposition of deltaically influenced sedimentation in the Roztocze Hills during the Campanian initially proposed by Remin et al. (2015a) and Walaszczyk and Remin (2015) and fully supports their general sedimentological and bathymetrical model (compare Fig. 2d). This bathymetric model and a shallow water position of the opoka facies (coastal environment) has been recently confirmed independently by Jurkowska et al. (2019a, b) and Machalski and Malchyk (2019).
In this respect, a continuously growing San Anticlinorium during the Late Cretaceous acted as a source area for the siliciclastics of the Roztocze Hills. In the present study, for the first time, we can directly demonstrate that these terrigenous-rich deltaic sediments interfinger with gaize and opoka variants located slightly northeast of the recognized Szozdy deltaic system. It clearly shows that this specific facies – opoka – should be considered as having been deposited in a shallow-water environment, at least in this specific case, in opposition to previous notions rooted in different tectonic and sedimentological models, indicating its rather deep origination. In the SW–NE transect perpendicular to the Szozdy deltaic system and the Łysogóry–Dobrogea Land (in a place of present-day San Anticlinorium), as defined here, the more carbonate-rich deposits, including chalk, were deposited in more offshore and deeper zones located to the northeast of the Szozdy deltaic system. The presence of such a deeper zone is confirmed by the discovery of contourites (Krzywiec et al., 2009, 2018): the contourites current must have operated along a regional slope located northeast of both the Łysogóry–Dobrogea Land and the Szozdy deltaic system (Fig. 12).
We might find a quite similar facies and structural situation in the Subhercynian Cretaceous Basin, which underwent inversion approximately at the same time as the Mid-Polish Trough. The vast majority of the Coniacian to Campanian deposits, dominated by sandy and marly deposits, were derived from adjacent source areas, i.e., an elevated Harz Mountains (Voigt et al., 2004, 2006, 2008; von Eynatten et al., 2008). The Coniacian storm-dominated deposits and Santonian deposits of a low-energy delta plain to a tidal-flat environment consisting of sands were deposited close to the areas elevated during inversion tectonics and pass into deeper facies in more offshore zones.
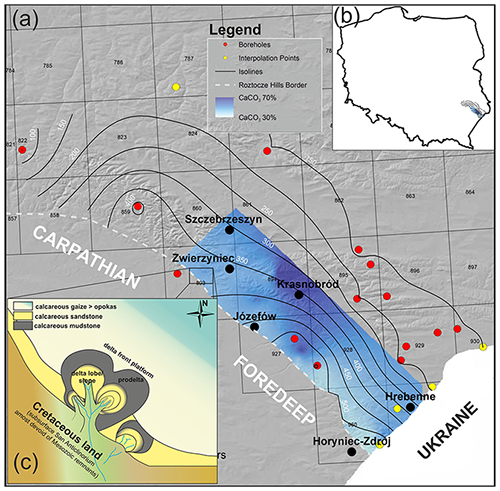
Figure 11(a) CaCO3 distribution pattern (blue area, compare Fig. 7) against the isolines of Campanian deposit thickness. Red dots: boreholes (compare Fig. 8); yellow dots: interpolation points; (b) general map of Poland with study area; (c) subaerial view of the Szozdy deltaic environment in relation to the distribution pattern of selected facies bordering the deltaic environment – not to scale. Squares with numbers represent the associated geological map sheets (https://geologia.pgi.gov.pl/, last access: 3 January 2022).
For several decades, our understanding of facies distribution in the Upper Cretaceous of extra-Carpathian Poland was heavily influenced by the paleotectonic model developed and proposed by Kutek and Głazek (1972). Those authors a priori synonymized the axial part of the Mid-Polish Trough (in the present day represented as the Mid-Polish Anticlinorium; Fig. 2b) with the most subsiding and deepest portion of the basin. It was consequently assumed that the facies deposited closest to the axial part of the Mid-Polish Trough (e.g., in the Roztocze Hills area) would represent the deepest sedimentary environment. As such, facies distributions and bathymetric interpretations were fit to this paleotectonic model (see also discussion in Walaszczyk and Remin, 2015). It is noteworthy, however, that Late Cretaceous mixed carbonate–siliceous facies elude easy environmental interpretation, and the adopted paleobathymetric model and the spatial distribution of particular facies (compare Fig. 2c) were not supported by any relevant sedimentological and/or biological indicators.
Nevertheless, the notion that opokas were largely of deep-water origin (Fig. 2c) has been widely accepted and for decades dominated thinking concerning the spatial distribution of facies and their relation to paleobathymetry (Leszczyński, 1997, 2010, 2012; Hakenberg and Świdrowska, 1998, 2001; Świdrowska and Hakenberg, 1999; Świdrowska, 2007; Świdrowska et al., 2008; Świerczewska-Gładysz, 2006). Accordingly, the Late Cretaceous opoka facies, the dominant facies of the Roztocze Hills, were considered as deep-water sediments until the present study, despite a considerable amount of terrigenous material (mainly sand-grained quartz, as well as clays). In light of the new data presented herein, this conception cannot be feasibly upheld, at least for the opoka facies of the Roztocze Hills.
5.5 Redistribution of terrigenous material
In a wider context, ocean currents (contourite currents) acting parallel to the shoreline of the identified Łysogóry–Dobrogea Land may have some influence on the redistribution of (mainly) the finest terrigenous material, pushing it away from the northern part of the Szozdy delta towards the southeastern direction (Figs. 11 and 12).
The existence of contourite currents operating along this putative landmass during the Campanian was revealed based on seismic profiles perpendicular to the landmass postulated here (Krzywiec et al., 2009, 2018) (Fig. 12). In general, their appearance within the basin might be related to the oceanographic, bathymetric condition, or enhanced tectonic activity (inversion), regulating the morphology of the seafloor (e.g., Rebesco et al., 2014). Similar structures, based on seismic data, have been also proved from the Danish Basin (e.g., Surlyk and Lykke-Andersen, 2007). Their recognition provides independent evidence for the paleo-circulation within the Polish Basin as based on surface data.
Intriguingly, the presence of currents flowing from the north and along the northern shore of the supposed paleobiogeographic barrier, interpreted here as a Łysogóry–Dobrogea Land (i.e., the area presently occupied by the San Anticlinorium), during the Coniacian and Santonian was suggested by Remin et al. (2016), who outlined its potential impact on paleocirculation, paleotemperature (as derived from oxygen isotopes), and the distribution of ammonite fauna. In further support of the existence of a paleobiogeographic barrier is the distribution pattern of belemnites during the early Maastrichtian (Remin, 2018).
The existence of contourite currents indicates that the Szozdy deltaic system was limited to the northeast by a regional slope on the sea bottom; currents flowing parallel to the strike redistributed terrigenous material towards the southeast. A potential manifestation of this current-driven redistribution of the finest terrigenous material may be pure clay deposits of approximately the same age located to the southeast of the Szozdy delta – that is, in the Horyniec Zdrój area (Figs. 11 and 12). This area is additionally characterized by the lowest amount of CaCO3 in the Campanian deposits of the Roztocze Hills and high terrestrial input.
Such a paleogeographic situation is, again, somewhat similar to the modern Mahakam deltaic system that borders the Makassar Trough: that is, a throughflow channel facilitating contourite currents (Shanmugam, 2017; Brackenridge et al., 2020), which redistributes material from the Mahakam prodelta to the south.
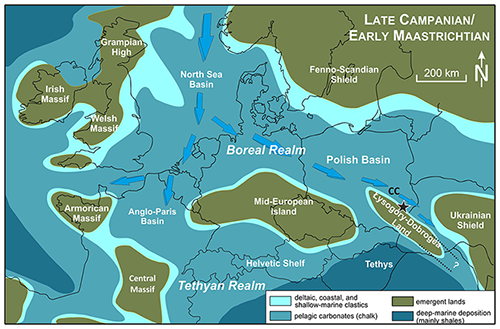
Figure 12Schematic paleogeographic map (compiled from Ziegler, 1990; Dadlez et al., 1998) and location of the Roztocze Hills and the Łysogóry–Dobrogea Land (see also Remin et al., 2016; Remin, 2018). The directions of ocean current flow are based on Remin et al. (2016) and Remin (2018); the presence of contourite currents (CC) is according to Krzywiec et al. (2009, 2018).
5.6 Drivers of accommodation space generation
Inversion-related tectonic instability along a deeply rooted fault zone (the southwestern boundary of the Roztocze Hills; Fig. 2a) led to the development of the Szozdy deltaic system. To the southwest of this fault zone, thus in the area of the subsurface San Anticlinorium (Fig. 2a and d), there existed a landmass during the Late Cretaceous – i.e., the Łysogóry–Dobrogea Land – that was a source area for the siliciclastics of the Szozdy delta. During the process of tectonic inversion, this area underwent uplift, which surely resulted in changes to detrital weathering, weatherability, erosion, and provenance. Correspondingly, on the other side of this fault zone (that is, to the northeast) the Upper Cretaceous strata of the present Roztocze Hills were deposited, including the cyclothems of the subaquatic delta front platform of the Szozdy deltaic system. This area, being part of an evolving marginal trough, experienced enhanced subsidence, which resulted in the deposition of thick Campanian strata, especially along this active fault zone (compare Figs. 8 and 11).
Subsidence of the Roztocze Hills area, on the one hand, and uplift and erosion of the Łysogóry–Dobrogea Land on the other (in the area of the modern San Anticlinorium) during the Late Cretaceous inversion could have conceivably driven the deltaic architecture, supplying additional accommodation space for the development of succeeding Szozdy delta cyclothems (Figs. 10 and 11). Furthermore, tectonically induced migration and avulsion of distributary channels led to changes in the local sedimentary environment, which resulted in a predictable repetition of Szozdy cyclothems.
5.7 Speculative comments on burial history and the rate of subsequent erosion
Since the paper of Kutek and Głazek (1972), the Holy Cross Mountains, and the San Anticlinorium to the southeast (Figs. 1b and 2a), have been traditionally included by most workers into the axial part of the former Mid-Polish Trough, which subsequently underwent inversion, uplift, erosion, and transformation into the Mid-Polish Anticlinorium. Apart from the concept of inversion tectonics onset itself, there was a general agreement that the axial parts of the Mid-Polish Anticlinorium (e.g., area of the recent-day Holy Cross Mountains and the San Anticlinorium) were deeply buried by thick Permian and Mesozoic sediments (e.g., Kutek and Głazek, 1972). Kutek and Głazek (1972) suggested that ca. 3000 m of sedimentary rocks have been removed by erosion from the axial part of the Mid-Polish Anticlinorium, especially from the Holy Cross Mountains and the San Anticlinorium (Figs. 1b and 2a). More recent estimations vary between 2000–2500 m (Resak et al., 2008; Narkiewicz et al., 2010). Similar values of overburden (1700–2300 m) were obtained by Łuszczak et al. (2020).
Since still little is known about possible Mesozoic overburden over the San Anticlinorium (this area is almost devoid of Mesozoic remnants; Figs. 1b and 2a), the heavy mineral assemblages from the Szozdy delta were analyzed to look for possible indicators of provenance. It provides intriguing data regarding the potential source rocks for the siliciclastics of the Szozdy deltaic system.
The heavy mineral assemblages from the Szozdy section exhibit a truly polycyclic nature. On the one hand, high values of the ZTR index (ascribed to ultrastable mineral phases), the high degree of roundness of (most) mineral grains, and the presence of some abrasive structures suggest multiple rounds of redeposition (Hubert, 1962; Mange and Wright, 2007; Garzanti, 2017; Garzanti and Andò, 2007, 2019). On the other hand, the presence of mineral groups weakly resistant to erosion – garnets, kyanites, and staurolites – that consist almost exclusively of angular crystals (> 70 %–80 %) with almost no oval and/or rounded representatives are inconsistent with repeated redeposition; instead, they support a multi-source provenance for the Szozdy deltaic deposits, as further elaborated below.
Accordingly, the above data suggest the presence of at least two distinct sources. The first yielded multi-recycled mineral phases dominated by minerals from the ZTR group, for which the source might be metapelites or metapsamites. The second, “fresh” source delivered angular-shaped crystals of garnets, kyanites, and staurolites, for which amphibolite facies rocks were the main source.
These data suggest that the source rocks for the heavy mineral assemblages of the Szozdy delta are represented by both (i) multi-recycled sedimentary rocks and (ii) freshly weathered metamorphic and igneous rocks. These characteristics would be rather difficult to obtain from the Mesozoic sedimentary cover of the San Anticlinorium (if it was even present at all), necessitating a search for other sources. A tempting hypothesis for the potential source rocks, although highly speculative, would be a comparison of mineral assemblages from the Szozdy delta to the Cambrian and Neoproterozoic rocks subcropping beneath the Miocene deposits of the Carpathian Foredeep, i.e., the recent San Anticlinorium, represented by metamorphosed (anchimetamorphic) flysch-type siliciclastics.
The interpretation presented herein implies the presence of a landmass area – that is, the Łysogóry–Dobrogea Land – in a place where, according to many previous interpretations, the deepest part of the Mid-Polish Trough was located. For the first time, clear and conclusive evidence for this iconoclastic paleogeographic and paleobathymetric model is provided, based on sedimentological data coupled with heavy mineral and palynofacies analyses. We recommend that the name Łysogóry–Dobrogea Land should be used, as it has priority (vide Samsonowicz, 1925).
The recognition of the Szozdy deltaic system in the supposedly axial part of the Mid-Polish Trough grants a unique perspective into the sedimentary environment, hydrodynamics, facies architecture, and bathymetric position of several Late Cretaceous facies. For instance, various styles of opoka facies are located adjacent to deltaic deposits, consistent with a shallow-water origin. A deeper sedimentary environment is suggested for the chalk facies.
Inversion-related tectonic instability along the deeply rooted fault zone at the southwestern boundary of the Roztocze Hills led to the development of the Szozdy deltaic system. Uplift of the present-day San Anticlinorium area during the Late Cretaceous inversion, on the one hand, and enhanced subsidence of the present-day area of the Roztocze Hills, on the other, rearranged the delta architecture, supplying additional accommodation space for the development of Szozdy delta cyclothems.
The Łysogóry–Dobrogea Land, located to the south and southwest of the Roztocze Hills – thus, where the San Anticlinorium is presently situated – is the only reasonable source for the siliciclastics of the Szozdy deltaic system. This area is currently almost completely devoid of Cretaceous and other Mesozoic remnants and is now hidden under the Miocene cover of the Carpathian Foredeep.
The geochemistry of tourmalines and garnets (compare also Cyglicki and Remin, 2016, 2018) may suggest that the strata subcropping beneath the Miocene series of the Carpathian Foredeep (to the southwest of the Roztocze Hills) were already emerged and eroding during the Late Cretaceous, at least to some degree.
The results obtained in this study and from previous research raise several crucial issues that should be addressed in the near future and furthermore allow us to formulate a working hypothesis that the modern San Anticlinorium was an elevated structural element during the Late Cretaceous. Such a hypothesis, although highly speculative, is supported by the initial geochemical data of heavy minerals we obtained, which might suggest that at least part of the material is derived from freshly weathered metamorphosed rocks. Further evidence and verification of these postulations would allow the following issues to be examined:
-
What was the source region for the Late Cretaceous Szozdy deltaic system and siliciclastics of the Roztocze Hills? Was it the Mesozoic cover of the San Anticlinorium? Or, were the Cambrian and Neoproterozoic rocks beneath the Miocene cover of the Carpathian Foredeep already eroding during the Cretaceous?
-
Did the San Anticlinorium constitute a homogeneous part (structurally and paleogeographically) of the Mid-Polish Trough during the Late Cretaceous (Santonian–Maastrichtian)?
-
In this context, what was the real nature of the southeastern extension of this trans-European sedimentary basin, the Mid-Polish Trough, during the Cretaceous?
-
Accordingly, what was the burial history of the San Anticlinorium, if burial had occurred at all?
Deciphering these crucial objectives, based on a revised paleofacies and paleobathymetric model, is of fundamental significance for understanding the Late Cretaceous paleotectonic evolution of the supposed southeastern edge of the former Mid-Polish Trough.
In turn, a precise recognition of the stratigraphic succession and facies distribution (work which is currently ongoing) in the Roztocze Hills will allow for the reliable identification of the main phases of terrigenous material input from the nearby landmass, along with the estimation of subsidence gradients and the identification of these events on high-resolution seismic profiles (also ongoing). In this framework, a reduction of the subsidence rate should lead to the progradation of the siliciclastic facies toward the northeast, thus trending from more proximal to more offshore zones.
Solving the issues outlined above, especially with regards to resolving whether or not the San Anticlinorium constitutes a structural part of the Mid-Polish Trough, may fundamentally change our understanding of regional geology and burial history, and more broadly speaking the paleogeography and paleobiogeography of central Europe during the Late Cretaceous, with far-reaching interpretative consequences.
Borehole and thickness data are stored in the Central Geological Database (CBDG; http://baza.pgi.gov.pl/, last access: 3 January 2022) of the Polish Geological Institute – Polish Research Institute (PIG-PIB).
ZR contributed to the idea, conceptualization, data compilation, discussion and validation, original draft, revision, and funding acquisition. MC contributed to the conceptualization, heavy minerals analyses, discussion and validation, and revision. MN contributed to the conceptualization, palynofacies analyses, discussion and validation, and revision.
The contact author has declared that neither they nor their co-authors have any competing interests.
Publisher’s note: Copernicus Publications remains neutral with regard to jurisdictional claims in published maps and institutional affiliations.
This article is part of the special issue “Inversion tectonics – 30 years later”. It is not associated with a conference.
Our thanks are extended to Bartosz Gościmski and Joanna Roszkowska-Remin for help during the fieldwork. Special thanks go to Robert Banaś, the chief forester of the Lubaczów district, and local forest officers who granted access to several outcrops and provided help in the field. Jordan Todes (University of Chicago, USA) kindly provided the linguistic improvement of the paper.
This research has been supported by the National Science Centre, Poland (grant no. UMO-2018/29/B/ST10/02947).
This paper was edited by Jonas Kley and reviewed by Aleksandra Stachowska, Thomas Voigt, and one anonymous referee.
Allen, G. P., Laurier, D., and Thouvenin, J.: Sediment distribution patterns in the modern Mahakam delta, Proc. Indonesian Petrol. Assoc., 5, 159–178, 1977.
Andò, S., Garzanti, E., Padoan, M., and Limonta, M.: Corrosion of heavy minerals during weathering and diagenesis: A catalog for optical analysis, Sediment. Geol., 280, 165–178, 2012.
Andò, S., Morton, A. C., and Garzanti, E.: Metamorphic grade of source rocks revealed by chemical fingerprints of detrital amphibole and garnet, Geol. Soc. Lond. Spec. Publ., 386, 351–371, 2013.
Błaszkiewicz, A.: Campanian and Maastrichtian ammonites of the Middle Vistula River valley, Poland: a stratigraphic and paleontological study, Prace Instytutu Geologicznego, 92, 1–63, 1980.
Brackenridge, R. E., Nicholson, U., Sapiie, B., Stow, D., and Tappin, D. R.: Indonesian Throughflow as a preconditioning mechanism for submarine landslides in the Makassar Strait, Geol. Soc. Lond. Spec. Publ., 500, 195–217, 2020.
Buła, Z. and Habryn, R.: Precambrian and Palaeozoic basement of the Carpathian Foredeep and the adjacent Outer Carpathians (SE Poland and western Ukraine), Ann. Soc. Geol. Pol., 81, 221–239, 2011.
Buła, Z., Byś, I., Florek, R., Habryn, R., Jachowicz, M., Kwarciński, J., Laskowicz, R., Liszka, B., Madej, K., Maksym, A., Markowiak, M., Pietrusiak, M., Probulski, J., Ryłko, W., Salwa, S., Sikora, R., Staryszak, G., Tabol-Wójcik, P., Tomaś, A., and Zacharski, J.: Geological-structural atlas of the Palaeozoic basement of the Outer Carpathians and Carpathian Foredeep, Polish Ministry of Environment, Warszawa, 104, 775–796, 2008.
Coleman, J. M. and Wright, L. D. (Eds): Modern river deltas: variability of processes and sand bodies, edited by: M. L. Broussard, Deltas, 2nd Edn., Houston Geological Society, 99–150, 1975.
Cyglicki, M. and Remin, Z.: Provenance of heavy minerals in the middle Campanian (Cretaceous) siliciclastic deposits of the Roztocze Hills, SE Poland, in: X Baltic Stratigraphic Conference, Chęciny, 28–29, 2016.
Cyglicki, M. and Remin, Z.: HRHMA (high-resolution heavy mineral analysis) zastosowana dla górno-kampańskich skałsilikoklastycznych krawędziowej części Roztocza Środkowego, SE Polska, Pokos, VII, 67, 2018.
Dadlez, R.: Mesozoic thickness pattern in the Mid-Polish Trough, Geol. Q., 47, 223–240, 2003.
Dadlez, R., Marek, S., and Pokorski, J. (Eds.): Geological map of Poland without Cenozoic deposits at 1:1 000 000 scale, Warszawa, Polish Geological Institute, 2000.
Dadlez, R., Narkiewicz, M., Stephenson, R. A. Visser, M. T. M., and van Wess, J. D.: Tectonic evolution of the Mid-Polish Trough: modelling implications and significance for central European geology, Tectonophysics, 252, 179–195, 1995.
Dadlez, R., Marek, S., and Pokorski, J. (Eds.): Palaeographical Atlas of the Epicontinental Permian and Mesozoic in Poland (1:2 500 000), Polish Geological Institute, Warszawa, Poland, 1998 (in Polish with English summary).
Downie, C., Hussain, M. A., and Williams, G. L.: Dinoflagellate cyst and acritarch associations in the Paleogene of southeast England, Geosci. Man., 3, 29–35, 1971.
Dubicka, Z., Peryt, D., and Szuszkiewicz, M.: Foraminiferal evidence for paleogeographic and paleoenvironmental changes across the Coniacian–Santonian boundary in western Ukraine, Palaeogeogr. Palaeocl., 401, 43–56, 2014.
Dziadzio, P. and Jachowicz, M.: Budowa podłoża utworów mioceńskich na SW od wyniesienia Lubaczowa, Przegląd Geologiczny, 44, 1124–1130, 1996.
Dziadzio, P., Maksym, A., and Olszewska, B.: Miocene deposition in the eastern part of the Carpathian Foredeep in Poland, Przegląd Geologiczny, 54, 413–420, 2006.
Eshet, Y., Almogi-Labin, A., and Bein, A.: Dinoflagellate cysts, paleoproductivity and upwelling systems: A Late Cretaceous example from Israel, Mar. Micropaleontol., 23, 231–240, 1994.
Galloway, W. E.: Process framework for describing the morphologic and stratigraphic evolution of deltaic depositional systems, in: Deltas, Models for Exploration, edited by: Broussard, M. L., Houston Geological Society, Houston, TX, 87–98, 1975.
Garzanti, E.: The maturity myth in sedimentology and provenance analysis, J. Sediment. Res., 87, 353–365, 2017.
Garzanti, E. and Andò, S.: Heavy mineral concentration in modern sands: implications for provenance interpretation, in: Heavy minerals in use, edited by: Mange, M. and Wright, D. T., Elsevier, 517–545, https://doi.org/10.1016/S0070-4571(07)58020-9, 2007.
Garzanti, E. and Andò, S.: Heavy minerals for Junior Woodchucks, Minerals, 9, 148, 1–25, 2019
Hakenberg, M. and Świdrowska, J.: Evolution of the Holy Cross segment of the Mid-Polish Trough during the Cretaceous, Geol. Q., 42, 239–262, 1998.
Hakenberg, M. and Świdrowska, J.: Cretaceous basin evolution in the Lublin area along the Teisseyre-Tornquist Zone (SE Poland), Ann. Soc. Geol. Pol., 71, 1–20, 2001.
Halamski, A. T.: Latest Cretaceous leaf floras from Southern Poland and Western Ukraine, Acta Paleontol. Pol., 58, 407–433, 2013.
Hardy, M. J. and Wrenn, J. H.: Palynomorph distribution in modern tropical deltaic and shelf sediments – Mahakam Delta, Borneo, Indonesia, Palynology, 33, 19–42, 2009.
Henry, D. J. and Guidotti, C. V.: Tourmaline as a petrogenetic indicator mineral: an example from the staurolite-grade metapelites of NW Maine, Am. Mineral., 70, 1–15, 1985.
Hubert, J. F.: A zircon-tourmaline-rutile maturity index and interdependence of the composition of heavy mineral assemblages with the gross composition and textures of sandstones, J. Sediment. Res., 32, 440–450, 1962.
Jaskowiak-Schoeneichowa, M. and Krassowska, A.: Paleomiąższości, litofacje i paleotektonika epikontynentalnej kredy górnej w Polsce, Geol. Q., 32, 177–198, 1988.
Jurkowska, A. and Barski, M.: Maastrichtian island in the Central European Basin-new data inferred from palynofacies analysis and inoceramid stratigraphy, Facies, 63, 1–20, 2017.
Jurkowska, A., Barski, M., and Worobiec, E.; The relation of a coastal environment to early diagenetic clinoptilolite (zeolite) formation-New data from the Late Cretaceous European Basin, Palaeogeogr. Palaeocl., 524, 166–182, 2019a.
Jurkowska, A., Świerczewska-Gładysz, E., Bąk, M., and Kowalik, S.: The role of biogenic silica in the formation of Upper Cretaceous pelagic carbonates and its palaeoecological implications, Cretaceous Res., 93, 170–187, 2019b.
Kamieński, M.: Przyczynek do znajomości kredy żurawieńskiej. Contribution a'la connaissance du facies Sablonneux des cauches cre'tace'es de Żurawno (Pologne), Kosmos, 50, 1408–1425, 1925.
Kiersnowski, H., Paul, J., Peryt, T. M., and Smith, D. B.: Facies, paleogeography, and sedimentary history of the Southern Permian Basin in Europe, in: The Permian of Northern Pangea, edited by: Scholle, P., Peryt, T. M., and Ulmer-Scholle, D., Vol. 2, Sedimentary Basins and Economic Resources, Springer, Berlin, 119–136, https://doi.org/10.1007/978-3-642-78590-0_7, 1995.
Kley, J. and Voigt, T.: Late Cretaceous intraplate thrusting in Central Europe: Effect of Africa-Iberia-Europe convergence, not Alpine collision, Geology, 36, 839–842, 2008.
Komar, P., D.: The entrainment, transport, and sorting of heavy minerals by waves and currents, in: Heavy minerals in use, edited by: Mange, M. and Wright, D. T., Elsevier, 3–48, https://doi.org/10.1016/S0070-4571(07)58001-5, 2007.
Kowalska, S., Kranc, A., Maksym, A., and Śmist, P.: Geology of the north-eastern part of the Carpathian Foredeep basement, the Lubaczów-Biszcza Region, Nafta-Gaz, 54, 158–178, 2000 (in Polish).
Krzywiec, P.: Miocene tectonic evolution of the Eastern Carpathian Foredeep Basin (Przemyśl–Lubaczów) in light of seismic data interpretation, Prace Państwowego Instytutu Geologicznego, 168, 249–276, 1999 (in Polish).
Krzywiec, P.: O mechanizmach inwersji bruzdy śródkowopolskiej – wyniki interpretacji danych sejsmicznych (On mechanism of the Mid-Polish Trough inversion), Biuletyn Państwowego Instytutu Geologicznego, 393, 135–166, 2000.
Krzywiec, P.: Mid-Polish Trough inversion – Seismic examples, main mechanisms and its relationship to the Alpine Carpathian collision, Continental collision and the tectonosedimentary evolution of forelands, European Geophysical Society Special Publication, 1, 151–165, 2002.
Krzywiec, P.: Structural inversion of the Pomeranian and Kuiavian segments of the Mid-Polish Trough–lateral variations in timing and structural style, Geol. Q., 50, 151–168, 2006.
Krzywiec, P.: Devonian–Cretaceous repeated subsidence and uplift along the Teisseyre–Tornquist zone in SE Poland—Insight from seismic data interpretation, Tectonophysics, 475, 142–159, 2009.
Krzywiec, P. and Stachowska, A.: Late Cretaceous inversion of the NW segment of the Mid-Polish Trough – how marginal troughs were formed, and does it matter at all?, Z. Dtsch. Ges. Geowiss., 167, 107–119, https://doi.org/10.1127/zdgg/2016/0068, 2016.
Krzywiec, P., Gutkowski, J., Walaszczyk, I., Wróbel, G., and Wybraniec, S.: Tectonostratigraphic model of the Late Cretaceous-inversion along the Nowe Miasto–Zawichost fault zone, SE Mid-Polish Trough, Kwartalnik Geologiczny, 53, 27–48, 2009.
Krzywiec, P., Peryt, T. M., Kiersnowski, H., Pomianowski, P., Czapowski, G., and Kwolek, K.: Permo-Triassic evaporites of the Polish Basin and their bearing on the tectonic evolution and hydrocarbon system, an overview, in: Permo-Triassic Salt Provinces of Europe, North Africa and the Central Atlantic: Tectonics and Hydrocarbon Potential, edited by: Soto, J. I., Flinch, J. F., and Tari, G., Elsevier, Amsterdam, 243–261, https://doi.org/10.1016/B978-0-12-809417-4.00012-4, 2017.
Krzywiec P., Stachowska A., and Stypa A.: The only way is up – on Mesozoic uplifts and basin inversion events in SE Poland, in: Mesozoic Resource Potential in the Southern Permian Basin, edited by: Kilhams, B., Kukla, P. A., Mazur, S., McKie, T., Mijnlieff, H. F., and van Ojik, K., Geol. Soc. Lond. Spec. Publ., 469, https://doi.org/10.1144/SP469.14, 2018.
Kutek, J. and Głazek, J.: The Holy Cross area, Central Poland, in the Alpine cycle, Acta Geol. Pol., 22, 603–652, 1972.
Kvale, E. P.: Tidal Constituents of Modern and Ancient Tidal Rhythmites: Criteria for Recognition and Analyses, in: Principles of Tidal Sedimentology, edited by: Davis Jr., R. A. and Dalrymple, R. W., Springer, 1–17, https://doi.org/10.1007/978-94-007-0123-6_1, 2012.
Leszczyński, K.: The Upper Cretaceous carbonate-dominated sequences of the Polish Lowlands, Geol. Q., 41, 521–532, 1997.
Leszczyński, K.: Lithofacies evolution of the Late Cretaceous basin in the Polish Lowlands, Biuletyn Państwowego Instytutu Geologicznego, 443, 33–54, 2010 (in Polish with English summary).
Leszczyński, K.: The internal geometry and lithofacies pattern of the Upper Cretaceous–Danian sequence in the Polish Lowlands, Geol. Q., 56, 363–386, 2012.
Leszczyński, K. and Dadlez, R.: Subsidence and the problem of incipient inversion in the Mid-Polish Trough based on thickness maps and Cretaceous lithofacies analysis – discussion, Przegląd Geologiczny, 47, 625–628, 1999 [in Polish with English summary].
Lewis, J., Dodge, J. D., and Powell, A. J.: Quaternary dinoflagellate cysts from the upwelling system offshore Peru, Hole 686B, ODP Leg 112, Proceedings of the Ocean Drilling Program, Scientific Results, 112, 323–328, 1990.
Łuszczak, K., Wyglądała, M., Śmigielski, M., Waliczek, M., Matyja, B. A., Konon, A., and Ludwiniak, M.: How to deal with missing overburden – Investigating exhumation of the fragment of the Mid-Polish Anticlinorium by a multi-proxy approach, Mar. Petrol. Geol., 114, 104–229, 2020.
Machalski, M. and Malchyk, O.: Relative bathymetric position of the opoka and chalk in the Late Cretaceous European Basin, Cretaceous Res., 102, 30–36, 2019.
Mange, M. A. and Maurer, H. F. W.: Heavy mineral in colour, Springer Science & Business Media, https://doi.org/10.1007/978-94-011-2308-2, 1992.
Mange, M. A. and Morton, A. C.: Geochemistry of heavy minerals, in: Heavy minerals in use, edited by: Mange, M. and Wright, D. T., Elsevier, 345–391, https://doi.org/10.1016/S0070-4571(07)58013-1, 2007.
Mange, M. A. and Wright, D. T.: High-resolution heavy mineral analysis (HRHMA): a brief summary, in: Heavy minerals in use, edited by: Mange M. and Wright D. T., Elsevier, 433–436, https://doi.org/10.1016/S0070-4571(07)58016-7, 2007.
Meres, Š.: Garnets – important information resource about source area and parental rocks of the siliciclastic sedimentary rocks, in: Conference “Cambelove dni 2008”, Abstract Book, edited by: Jurkovič, L., Comenius University, Bratislava, 37–43, 2008 (in Slovak with English abstract).
Narkiewicz, M. and Petecki, Z.: Basement structure of the Paleozoic Platform in Poland, Geol. Q., 61, 502–520, 2017.
Narkiewicz, M., Resak, M., Littke, R., and Marynowski, L.: New constraints on the Middle Palaeozoic to Cenozoic burial and thermal history of the Holy Cross Mts. (Central Poland): results from numerical modelling, Geol. Acta, 8, 189–205, 2010.
Narkiewicz, M., Maksym, A., Malinowski, M., Grad, M., Guterch, A., Petecki, Z., Probulski, J., Janik, T., Majdański, M., Środa, P., Czuba, W., Gaczyński, E., and Jankowski, L.: Transcurrent nature of the Teisseyre–Tornquist Zone in Central Europe: results of the POLCRUST-01 deep reflection seismic profile, Int. J. Earth Sci., 104, 775–796, 2015.
Niechwedowicz, M., Cyglicki, M., and Remin, Z.: Zmiany zespołów cyst dinoflagellata z osadów deltowych środkowego kampanu Roztocza, SE Polska – implikacje środowiskowe, in: III Polski Kongres Geologiczny, Wrocław, 267–268, 2016.
Nielsen, S. B. and Hansen, D. L.: Physical explanation of the formation and evolution of inversion zones and marginal troughs, Geology, 28, 875–878, 2000.
Nowak, J.: Przyczynek do znajomości kredy Lwowsko-Rawskiego Roztocza, Kosmos, 32, 160–169, 1907.
Nowak, J.: Spostrzeżenia w sprawie wieku kredy zachodniego Podola, Kosmos, 33, 279–285, 1908.
Olde, K., Jarvis, I., Uličný, D., Pearce, M. A., Trabucho-Alexandre, J., Čech, S., Gröcke, D. R., Laurin, J., Švábenická, L., and Tocher, B. A.: Geochemical and palynological sea-level proxies in hemipelagic sediments: A critical assessment from the Upper Cretaceous of the Czech Republic, Palaeogeog. Palaeocl., 435, 222–243, 2015.
Omran, E. F.: The Nile delta: Processes of heavy mineral sorting and depositional patterns, in: Heavy minerals in use, edited by: Mange M. and Wright D. T., Elsevier, 49–74, https://doi.org/10.1016/S0070-4571(07)58002-7, 2007.
Pharaoh, T. C., Dusar, M, Geluk, M. C., Kockel, F., Krawczyk, C. M., Krzywiec, P., Scheck-Wenderoth, M., Thybo, H., Vejbæk, O. V., and Van Wees, J. D.: Tectonic evolution, in: Petroleum Geological Atlas of the Southern Permian Basin Area, edited by: Doornenbal, J. C. and Stevenson, A. A., European Association of Geoscientists and Engineers (EAGE), Houten, the Netherlands, 25–57, https://doi.org/10.3997/2214-4609.20145798, 2010.
Pasternak, S. I.: Biostratygrafiya kreydovykh vidkladiv Volyno-Podilskoi plyty, Akademia Nauk Ukrainskoi RSR, Kiev, 3–98, 1959.
Pasternak, S. I., Gavrylyshyn, V. I., Ginda, V. A., Kotsyubinsky, S. P., and Senkovskyi, Y. M.: Stratygrafia i fauna kredowych vidkladiv zachodu Ukrainy, Naukova Dumka, Kiev, 1–272, 1968.
Pasternak, S. I., Senkovskyi, Y. M., and Gavrylyshyn, V. I.: Volyno-Podillya u kreydovomu periodi, Naukova Dumka, Kiev, 3–258, 1987.
Powell, A. J., Dodge, J. D., and Lewis, J.: Late Neogene to Pleistocene palynological facies of the Peruvian continental margin upwelling, Leg 112, Proceedings of the Ocean Drilling Program, Scientific Results, 112, 297–321, 1990.
Powell, A. J., Lewis, J., and Dodge, J. D.: The palynological expressions of post-Palaeogene upwelling: a review, in: Upwelling Systems: Evolution Since the Early Miocene, edited by: Summerhayes, C. P., Prell, W. L., and Emeis, K. C., Geol. Soc. Spec. Publ., 64, 215–226, 1992.
Pożaryski, W.: Kreda, in: Regionalna geologia Polski, 2, edited by: Książkiewicz, M. and Dżułyński, S., Polskie Towarzystwo Geologiczne, Państwowe Wydawnictwo Naukowe, Kraków, 1956.
Pożaryski, W.: Zarys stratygrafii i paleogeografii na Niżu Polskim, Prace Instytutu Geologicznego, 30, 377–418, 1960.
Pożaryski, W.: Kreda, in: Atlas geologiczny Polski. Zagadnienia stratygraficzno-facjalne, Instytut Geologiczny, 10, 1962.
Pożaryski, W.: Tectonics, Part 1. Polish Lowlands, in: Geology of Poland IV, edited by: Pożaryski, W., Wydawnictwa Geologiczne, Warszawa, 2–34, 1974.
Preston, J., Hartley, A., Mange-Rajetzky, M., Hole, M., May, G., Buck, S., and Vaughan, L.: The provenance of Triassic continental sandstones from the Beryl Field, northern North Sea: mineralogical, geochemical, and sedimentological constraints, J. Sediment. Res., 72, 18–29, 2002.
Rebesco, M., Hernández-Molina, F. J., Van Rooij, D., and Wåhlin, A.: Contourites and associated sediments controlled by deep-water circulation processes: State-of-the-art and future considerations, Mar. Geol., 352, 111–154, 2014.
Remin, Z.: The Belemnella stratigraphy of the Campanian–Maastrichtian boundary; a new methodological and taxonomic approach, Acta Geol. Pol., 62, 495–533, 2012.
Remin, Z.: The Belemnitella stratigraphy of the Upper Campanian–basal Maastrichtian of the Middle Vistula section, central Poland, Geol. Q., 59, 783–813, 2015.
Remin, Z.: Understanding coleoid migration patterns between eastern and western Europe–belemnite faunas from the upper lower Maastrichtian of Hrebenne, southeast Poland, Cretaceous Res., 87, 368–384, 2018.
Remin, Z., Cyglicki, M., Cybula, M., and Roszkowska-Remin, J.: Deep versus shallow? Deltaically influenced sedimentation and new transport directions – case study from the Upper Campanian of the Roztocze Hills, SE Poland, in: Proceedings of the 31st IAS Meeting of Sedimentology, Kraków, Poland, p. 438, 2015a.
Remin, Z., Machalski, M., and Jagt, J. W. M.: The stratigraphically earliest record of Diplomoceras cylindraceum (heteromorph ammonite) – implications for Campanian/Maastrichtian boundary definition, Geol. Q., 59, 843–848, 2015b.
Remin, Z., Gruszczyński M., and Marshall J. D.: Changes in paleo-circulation and the distribution of ammonite faunas at the Coniacian–Santonian transition in central Poland and Western Ukraine, Acta Geol. Pol., 66, 107–124, 2016.
Resak, M., Narkiewicz, M., and Littke, R.: New basin modelling results from the Polish part of the Central European Basin system: implications for the Late Cretaceous–Early Paleogene structural inversion, Int. J. Earth Sci., 97, 955–972, 2008.
Roberts, H. H. and Sydow, J.: Late Quaternary stratigraphy and sedimentology of the offshore Mahakam delta, East Kalimantan (Indonesia), in: Tropical Deltas of Southeast Asia – Sedimentology, Stratigraphy, and Petroleum Geology, edited by: Sidi, F. H., Nummedal, D., Imbert, P., Darman, H., and Posamentier, H. W., SEPM Special Publication, 76, 125–145, 2003.
Rogala, W.: O stratygrafii utworów kredowych Podola, Kosmos, 34, 1160–1164, 1909.
Ryan, P. D., Mange, M. A., and Dewey, J. F.: Statistical analysis of high-resolution heavy minerals stratigraphic data from ordovician of western Ireland and its tectonic consequences, in: Heavy minerals in use, edited by: Mange M. and Wright D. T., Elsevier, 465–489, https://doi.org/10.1016/S0070-4571(07)58018-0, 2007.
Samsonowicz, J.: Szkic geologiczny okolic Rachowa nad Wisłą, Sprawozdania Państwowego Instytutu Geologicznego, 3, 45–118, 1925.
Shanmugam, G.: Contourites: Physical oceanography, process sedimentology, and petroleum geology, Petroleum exploration and development, 44, 183–216, 2017.
Storms, J. E., Hoogendoorn, R. M., Dam, R. A., Hoitink, A. J. F., and Kroonenberg, S. B.: Late-holocene evolution of the Mahakam delta, East Kalimantan, Indonesia, Sedimentary Geology, 180, 149–166, 2005.
Surlyk, F. and Lykke-Anderson, H.: Contourite drifts, moats and channels in the Upper Cretaceous chalk of the Danish Basin, Sedimentology, 54, 405–422, https://doi.org/10.1111/j.1365-3091.2006.00842.x, 2007.
Świdrowska, J.: Kreda w regionie lubelskim-sedymentacja i jej tektoniczne uwarunkowania, Biuletyn Instytutu Geologicznego, 422, 63–78, 2007.
Świdrowska, J. and Hakenberg, M.: Subsydencja i początki inwersji bruzdy śrdódpolskiej na podstawie analizy map miąższości i litofacji osadów górnokredowych, Przegląd Geologiczny, 47, 61–68, 1999.
Świdrowska, J., Hakenberg, M., Poluhtovič, B., Seghedi, A., and Višnâkov, I.: Evolution of the Mesozoic basins on the southwestern edge of the East European Craton (Poland, Ukraine, Moldova, Romania), Studia Geologica Polonica, 130, 3–130, 2008.
Świerczewska-Gładysz, E.: Late Cretaceous siliceous sponges from the Middle Vistula River Valley (Central Poland) and their palaeoecological significance, Ann. Soc. Geol. Pol., 76, 227–296, 2006.
Turner, G. and Morton, A. C.: The effects of burial diagenesis on detrital heavy mineral grain surface textures, in: Heavy minerals in use, edited by: Mange M. and Wright D. T., Elsevier, 393–412, https://doi.org/10.1016/S0070-4571(07)58014-3, 2007.
Tyson, R. V.: Palynofacies analysis, in: Applied micropaleontology, edited by: Jenkins, D. G., Kluwer Academic Publishers, Dordrecht, the Netherlands, 153–191, 1993.
Tyson, R. V.: Sedimentary organic matter. Organic facies and palynofacies, Chapman and Hall, London, 615 pp., https://doi.org/10.1007/978-94-011-0739-6, 1995.
Velbel, M. A.: Bond strength and the relative weathering rates of Simple orthosilicates, Amer. J. Sci. 299, 679–696, 1999.
Velbel, M. A.: Surface textures and dissolution processes of heavy minerals in the sedimentary cycle: examples from pyroxenes and amphiboles, in: Heavy minerals in use, edited by: Mange M. and Wright D. T., Elsevier, 113–150, https://doi.org/10.1016/S0070-4571(07)58004-0, 2007.
Velbel, M. A., Basso Ch. L., and Zieg, M. J.: The natural weathering of staurolite: crystal-surface textures, relative stability, and the rate-determining step, American J. Sc. 296, 453–472, 1996.
Velbel, M. A., McGuire, J. T., and Madden, A. S.: Scanning electron microscopy of garnet from southern Michigan soils: etching rates and inheritance of pre-glacial and pre-pedogenic grain-surface textures, in: Heavy minerals in use, edited by: Mange M. and Wright D. T., Elsevier, 413–432, https://doi.org/10.1016/S0070-4571(07)58015-5, 2007.
Voigt, T., von Eynatten, H., and Franzke, H.-J.: Late Cretaceous unconformities in the Subhercynian Cretaceous Basin (Germany), Acta Geol. Pol., 54, 675–696, 2004.
Voigt, T., Wiese, F., von Eynatten, H., Franzke, H.-J., and Gaupp, R.: Facies evolution of syntectonic Upper Cretaceous Deposits in the Subhercynian Cretaceous Basin and adjoining areas (Germany), Z. Dt. Geol. Ges., 157, 203–244, 2006.
Voigt, T., Reicherter, K., von Eynatten, H., Littke, R. Voigt, S., and Kley, J.: Sedimentation during basin inversion, in: Dynamics of complex sedimentary basins, The example of the Central European Basin System, edited by: Littke, R., Bayer, U., Gajewski, D., and Nelskamp, S., 211–232, 2008.
Voigt, T., Kley, J., and Voigt, S.: Dawn and dusk of Late Cretaceous basin inversion in central Europe, Solid Earth, 12, 1443–1471, https://doi.org/10.5194/se-12-1443-2021, 2021.
von Eynatten, H., Voigt, T., Meier, A., Franzke, H.-J., and Gaupp, R.: Provenance of the clastic Cretaceous Subhercynian Basin fill: constraints to exhumation of the Harz Mountains and the timing of inversion tectonics in the Central European Basin, Int. J. Earth Sci., 97, 1315–1330, 2008.
Walaszczyk, I.: Turonian through Santonian deposits of the Central Polish Upland; their facies development, inoceramid paleontology and stratigraphy, Acta Geol. Pol., 42, 1–122, 1992.
Walaszczyk, I.: Inoceramids and inoceramid biostratigraphy of the Upper Campanian to basal Maastrichtian of the Middle Vistula River section, central Poland, Acta Geol. Pol., 54, 95–168, 2004.
Walaszczyk, I. and Remin, Z.: Kreda obrzeżenia Gór Świętokrzyskich, Przewodnik LXXXIV Zjazdu Polskiego Towarzystwa Geologicznego, Chęciny, September 9–11, 41–50, 2015.
Walaszczyk, I., Dubicka, Z., Olszewska-Nejbert, D., and Remin, Z.: Integrated biostratigraphy of the Santonian through Maastrichtian (Upper Cretaceous) of extra-Carpathian Poland, Acta Geol. Pol., 66, 313–350, 2016.
Wall, D., Dale, B., Lohmann, G. P., and Smith, W. K.: The environmental and climatic distribution of dinoflagellate cysts in modern marine sediments from regions in the North and South Atlantic Oceans and adjacent seas, Mar. Micropaleontol., 2, 121–200, 1977.
Wright, W. I.: The composition and occurrence of garnets, Am. Mineral., 23, 436–449, 1938.
Wyrtki, K.: Indonesian throughflow and the associated pressure gradient, J. Geophys. Res.-Ocean., 92, 12941–12946, 1987.
Ziegler, P. A.: Geological Atlas of Western and Central Europe, 2nd Edn. Shell Internationale Petroleum Maatschappij, The Hague, Geol. Soc., Lond., 1990.
Żelaźniewicz, A., Buła, Z., Fanning, M., Seghedi, A., and Żaba, J.: More evidence on Neoproterozoic terranes in southern Poland and southeastern Romania, Geol. Q., 53, 93–124, 2009.
Żelaźniewicz, A., Aleksandrowski, P., Buła, Z., Karnkowski, P., Konon, A., Oszczypko, N., Ślączka, A., Żaba, J., and Żytko, K.: Regionalizacja tektoniczna Polski, 1–60, Komitet Nauk Geologicznych, Wrocław, 2011.
- Abstract
- Introduction
- The current state of the art: a short story of two contrasting theories
- Regional setting
- Results
- Interpretation and discussion
- Summary
- Current challenges and future roadmap
- Data availability
- Author contributions
- Competing interests
- Disclaimer
- Special issue statement
- Acknowledgements
- Financial support
- Review statement
- References
- Abstract
- Introduction
- The current state of the art: a short story of two contrasting theories
- Regional setting
- Results
- Interpretation and discussion
- Summary
- Current challenges and future roadmap
- Data availability
- Author contributions
- Competing interests
- Disclaimer
- Special issue statement
- Acknowledgements
- Financial support
- Review statement
- References