the Creative Commons Attribution 4.0 License.
the Creative Commons Attribution 4.0 License.
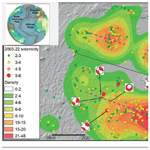
Offshore seismicity clusters in the West Iberian Margin illustrated by 2 decades of events
Gabriela Fernández-Viejo
Carlos López-Fernández
Patricia Cadenas
An analysis of 2 decades (2003–2022) of seismicity recorded by the Spanish and Portuguese seismic networks along the West Iberian passive margin has resulted in a better understanding of the distribution of moderate seismic activity in this intraplate submarine area. The study provides a precise trend of specific alignments inferred from the density maps of seismicity, giving an accurate depiction of event distribution along two wide stripes that extend for 700 km through the ocean floor in the WNW–ESE direction. These bands are parallel to the Africa–Eurasia plate boundary but are distinctly separated from its related seismicity by approximately 300 and 700 km, respectively. This is a sufficient distance to be considered intraplate activity. When trying to relate this seismicity to structural and geophysical features, a conclusive picture does not emerge. The earthquakes occur indiscriminately across thinned continental, hyperextended, and exhumed mantle rift domains. They fade out in proximity to undisputed oceanic crust, but some events extend beyond. The hypocentral depths signal a considerable number of events nucleating in the upper mantle. The focal mechanisms, although scarce, are predominantly strike-slip. Considering these observations, hypotheses ranging from subduction initiation and development of strained corridors to local structures of the margin are discussed in order to explain this relatively anomalous seismicity. However, some of them lack convincing arguments, while others are too vague. None of them are flawless, suggesting that several factors may be at play. Despite being one of the most probed passive margins in the world, the present geodynamic status of the West Iberian Margin manifested in its modern seismicity remains unknown. Interpreting these data within a global tectonic plate framework, together with the potential addition of seafloor seismometers, may provide the key to understanding this activity along one of the most archetypical margins of the Atlantic Ocean.
- Article
(7081 KB) - Full-text XML
- BibTeX
- EndNote
1.1 Physiographic aspects and brief geological history of the West Iberian Margin
The West Iberian Margin (WIM) is an 850 km long passive margin parallel to the N–S-trending rectilinear coast of western Iberia that resulted from tectonic evolution marked by a multiphase rifting history (Pinheiro et al., 1996; Péron-Pinvidic et al., 2007; Tucholke et al., 2007; Alves et al., 2009; Pereira et al., 2016; Granado et al., 2021). Currently, the dynamics of the margin are influenced by two significant plate limits. To the west lies the mid-Atlantic ridge (MAR) constructive plate boundary. Meanwhile, to the south, the margin is impacted by the complex NW Africa–SW Eurasia plate limit, known as the Azores–Gibraltar fault zone (AGFZ) (Fig. 1). To the north, the West Iberian Margin (WIM) adjoins the E–W-trending southern Biscay passive margin, which is located to the south of the extinct Bay of Biscay ridge.
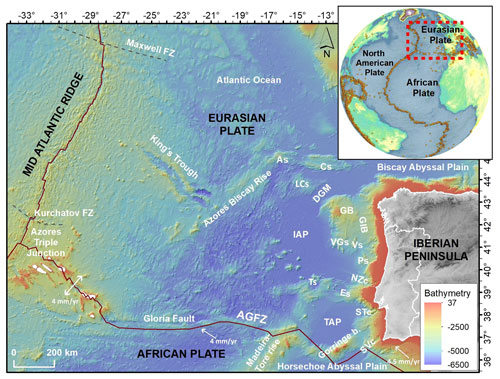
Figure 1Study area. The WIM and its main physiographic features including their western (mid-Atlantic ridge – MAR, Eurasia–America) and southern (Azores–Gibraltar fault zone – AGFZ, Eurasia–Africa) plate boundaries. GB: Galicia Bank, IAP: Iberian Abyssal Plain, GIB: Galicia Interior Basin, VGs: Vasco de Gama seamount, Vs: Vigo seamount, Ps: Porto seamount, NZc: Nazaré canyon, Es: Extremadura Spur, STc: Setubal canyon, TAP: Tagus Abyssal Plain, SVc: San Vicente canyon, FZ: fracture zone (Kurchatov). Cs: Charcot seamount, As: Atlantic seamount.
Along the WIM, the 30–85 km wide continental platform is noticeably intersected by deep E–W- to NE–SW-trending canyons, namely Nazaré (NZc), Setubal (STc), and San Vicente (SVc) (Fig. 1). Northwards of NZc, 200 km from the coast and separated from the platform by the Galicia Interior Basin (GIB), the Galicia Bank (GB) stands out, which is an elevated area with a relief of over 3500 m, along with other minor topographic seamounts. Westwards of the GB, the Iberian Abyssal Plain (IAP) emerges as an expansive and flat ocean floor that reaches a depth of 5300 m. As we move towards the MAR, the surface becomes considerably rough, indicative of oceanic crust. To the west of the IAP, the prominent Kings Trough (KT) is a 450 km long and WNW–ESE-trending scar in the oceanic floor (Fig. 1) (Srivastava and Roest, 1992), which consists of a central depression flanked by ridges. It is proposed that this feature formed from 56 to 25 Ma during Mesozoic rifting along the crest of a hotspot, resulting in an aseismic ridge which represented the plate boundary between Iberia and Eurasia. This boundary shifted and was active along the Bay of Biscay between 49 and 36 Ma, extending eastwards along the Pyrenees (Roest and Srivastava, 1991), and finally moved to its present location along the AGFZ at chron 13 (Macchiavelli et al., 2017). Between NZc and STc, the Extremadura Spur (Es) separates the IAP from the Tagus Abyssal Plain (TAP), a 200 km wide flat area situated at 5000 m depth. The TAP is limited to the west by the Madeira Tore Rise and to the southeast by the Gorringe Bank, one of the principal seismogenic sources in the region. The presence of areas of relief has been associated with N–S normal faults and NE–SW thrusts (Boillot et al., 1988; Pinheiro et al., 1996; Álvarez-Marrón, et al., 1997; Vázquez et al., 2008; Duarte et al., 2013; Sallarès et al., 2013; Martínez-Loriente et al., 2013), which in turn can be related to margin reactivation during the Mesozoic and the Paleogene (e.g., Jiménez-Munt and Negredo, 2013). Furthermore, the Extremadura Spur and related reliefs are connected to extensive Late Cretaceous alkaline magmatic occurrences (Pereira et al., 2016; Escada et al., 2022).
The mid-Atlantic ridge (MAR), a constructive plate boundary situated about 1500 km west of the WIM, separates the North American Plate from the Eurasian Plate. With a slow spreading rate, it accretes at a rate of 24–26 mm yr−1 at 43∘ N (Somoza et al., 2019). Unlike other areas of the North Atlantic, the MAR is remarkably devoid of significant transform faults, spanning over 1400 km without any such faults between 40.6 and 43.6∘ N and between 43.6 and 47.6∘ N, except for short ones with negligible displacement. This unique characteristic of the ridge in this section of the North Atlantic contrasts with its displacement by relevant transform faults both to the south and north of the WIM (Fig. 1).
The AGFZ plate boundary that limits the WIM southwards is structurally much more complex. The Southwest Iberian Margin (SWIM) faults (Zitellini et al., 2009; Martínez-Loriente et al., 2013) connect the Gloria Fault to the eastern part of the Africa–Europe plate boundary (Fig. 1). To the east of the Strait of Gibraltar, the plate boundary is defined by a right-lateral transpressive shear one (Morel and Meghraoui, 1996). In a recent seismic study Gomez de la Peña et al. (2022) interpret long and active faults accommodating most of the deformation in the western Mediterranean. The kinematic evolution of the Iberian margin during the Atlantic opening is well determined by magnetic anomalies after the Cretaceous normal polarity superchron (Roest and Srivastava, 1991). However, the early rift-to-drift seafloor evolution from 200 to 83 Ma remains controversial, mainly due to the dual assignment of the J anomaly either to a real isochron (e.g., Barnett-Moore et al., 2016) or to a polygenic magmatic event right before oceanic inception (e.g., Nirrengarten et al., 2017).
1.2 Crustal structure and domains of the WIM
The WIM fits into the category of a non-volcanic passive margin with a narrow platform (Lymer and Reston, 2022). It developed conjugate to the Newfoundland margin during Mesozoic rifting (Péron-Pinvidic and Manatschal, 2009). Its crustal structure, although debated, is formed by a series of structural domains from east to west (e.g., Péron-Pinvidic and Manatschal, 2009; Druet et al., 2019; Merino et al., 2021) and includes a Variscan basement (Schulmann et al., 2022). The segmentations observed within the WIM resulted from the northward migration of extension and successive Late Jurassic to Early Cretaceous extensional phases (e.g., Malod and Mauffret, 1990; Péron-Pinvidic et al., 2007; Tucholke et al., 2007; Merino et al., 2021).
Following the onset of break-up within the Pangea supercontinent 200 Myr ago, the pre-Jurassic template of the North Atlantic was subjected to three main phases of rifting that took place from the Late Triassic to Early Jurassic, from the Late Jurassic to Early Cretaceous, and during the mid-Cretaceous. The different rifting stages led to a system of tilted blocks limited by westward-dipping extensional detachment faults (Lymer and Reston, 2022), which show a N–S orientation in the north and variable trends in the south. At the end of rift-related deformation, during the Late Cretaceous, the WIM experienced continental break-up (Soares et al., 2012; King and Welford, 2022), leading to irregular regions of exhumed and serpentinized mantle (Afilhado et al., 2008; Merino et al., 2021; Grevemeyer et al., 2022). The onset of seafloor spreading occurred at least in two pulses, the first one at the end of the Jurassic between the Grand Banks and the TAP and the second one during the Early Cretaceous between the IAP and the Grand Banks, following the temporal northward opening of the Atlantic Ocean.
As a consequence of the later Alpine orogeny that formed the actual reliefs on land, there was an important compressive deformation, which produced the reactivation of some extensional faults as thrusts or as transpressive strike-slip faults (e.g., Pereira et al., 2011; Martínez-Loriente et al., 2013; Ramos et al., 2016; Terrinha et al., 2019). The Cenozoic deformation abruptly terminates against the ocean–continent transition (OCT).
Published works investigating the Mesozoic rift domains in the WIM distinguish two sectors. In the northern sector, the continental hyperextended crust stretches for more than 300 km and the exhumed mantle domain extends westwards of the GB (Druet et al., 2019). In the southern sector, contrastingly, the hyperextended crust extends for 200 km before reaching the same transition (Pedrera et al., 2017). The OCT extends between 12∘10′ W and 12∘30′ W in the IAP (Whitmarsh et al., 1990), and it is interpreted to extend 10∘ N for 130 km until the Extremadura Spur. According to Grevemeyer et al. (2022), the OCT runs between 12.5 and 13∘ W in the TAP, while Merino et al. (2021) locate the OCT between 13 and 13.5∘ W.
Crustal thickness based on refraction profiles north of the Extremadura Spur report thicknesses that shallow from almost 30 km close to the coast to 15 km in the ocean side with a quick thinning happening in 50 km of extension (Dean et al., 2000). Based on seismic-derived gravity modeling, Amigo Marx et al. (2022) differentiate an 18 km thick proximal domain, a <18 km thick extended domain, and a <7 km thick hyperextended domain. The exhumed mantle domain has been drilled at a serpentinite ridge (Boillot et al., 1988). Westwards of the Extremadura Spur, a thin lower crust has been interpreted beneath a dense upper crust. The thickness of the crust decreases quickly towards the west, while it does so gradually towards the north, creating two distinct domains. The first one is located near the continent and is characterized by a thin and light crust, while the second one is further away and has a denser and thicker crust (Amigo Marx et al., 2022).
Using seismic data, wells, and gravity data, Granado et al. (2021) modeled a more complex 3D crustal-scale structure of the WIM with two necking domains along the GIB and the deep Galicia basin in the west of the Galicia Bank, suggesting two rift directions. The GIB encompasses localized regions of significantly thinned crust that are segmented by NE–SW-trending transfer zones, and it has been interpreted as a failed rift (Murillas et al., 1990; King et al., 2020). The GB is a 15–20 km thick block of continental crust with relatively low thinning (Péron-Pinvidic and Manatschal, 2009; King et al., 2020). The outer necking zone merges southwards with the eastern one following a NW–SE orientation.
The limit between the northern and central WIM follows the NW–SE orientation that is observed by Granado et al. (2021) in their Bouguer gradient anomaly maps THD (total horizontal derivative) and dZ (vertical derivative). According to these authors, it relates to the Variscan fabric onshore, suggesting unrecognized transfer structures to explain the transition. A similar transfer zone SW of the GB was suggested earlier (Pinheiro et al., 1992; Whitmarsh et al., 1993) based on the analysis of magnetic anomalies and named the Figueira fracture zone, which would represent an original fracture zone born at the time of initiation of seafloor spreading.
So, within the general variations of the WIM, we can consider in our study area two of the three WIM segments from north to south: the Galicia margin that includes the GB and IAP as the northern segment and the central segment that includes the Extremadura Spur and the TAP. The seismicity related to the third and southernmost segment of the WIM, the so-called Southwest Iberian Margin, is out of the scope of this study, as it is considered to be related to the AGFZ plate boundary (Buforn et al., 1988).
1.3 Stress state, seismicity, and kinematics in the WIM
Seismicity is the expression of active stresses within plates, and these forces are intimately linked to kinematics. The so-called Iberian Plate takes its name from periods during which the Iberian Peninsula and part of its margins effectively acted as an independent minor tectonic plate between the surrounding and larger European and African plates, for instance from the late Eocene (chron 18, 42 Ma) to early Miocene (chron 6c, 24 Ma) (Roest and Srivastava, 1991). In earlier periods, Iberia was attached to Africa, having a plate boundary running in the northern part through the Bay of Biscay and the actual Pyrenees that subsequently moved in solidarity with Europe. The presence of thick post-orogenic and post-tectonic units offshore, sealing the Biscay accretionary wedge (Álvarez-Marrón et al., 1997; Fernández-Viejo et al., 2012), among other things, confirms that Iberia is now part of the Eurasian Plate without discussion. Nevertheless, Iberian kinematics have been a cause of study and controversy for a long time. The M sequence of magnetic anomalies along the Newfoundland margin and WIM forms one of the primary constraints for plate kinematic reconstructions characterizing the motion of Iberia during the Mesozoic (Vissers and Mejer, 2012; Sibuet et al., 2004; Barnett-Moore et al., 2016, and references therein). However, the validity of these anomalies has been questioned (Bronner et al., 2011). The difficulty in distinguishing different crustal types only adds uncertainty (Granado et al., 2021, and references therein). The seafloor spreading history of the western and northern margins of Iberia has been described involving successive jumps of the plate boundary between Iberia, Europe, and Africa from north (Bay of Biscay axis, and KT) to south (AGFZ) (Roest and Srivastava, 1991; Macchiavelli et al., 2017).
Complexity in kinematics is normally inversely proportional to the size of the plate. Therefore, numerous models of Iberian kinematics have been published in the last decades, the most modern ones approaching the problem through deformable plate tectonic models (King and Welford, 2022). These have provided insight into the kinematic role of continental blocks and their interplay with large and micro-tectonic plates during the formation of the North Atlantic rifted margins (e.g., Nirrengarten et al., 2018; Peace et al., 2019; King and Welford, 2022).
Macchiavelli et al. (2017) indicate a spreading rate of 23 mm yr−1 north of 40∘ W latitude and 20.5 mm yr−1 south of 38∘ W as well as 2 mm yr−1 of N–S convergence between Iberia and Africa since the Miocene. The earlier partitioning of convergence between north and south became totally accommodated by the southern boundary from the early Miocene onwards. The total convergence oscillates between 3 and 6 mm yr−1 (Fernandes et al., 2003; Nocquet, 2012; Serpelloni et al., 2004). It is characterized around the Azores archipelago by a NNE–SW extension that passes to the east to a dextral strike-slip border along the Gloria Fault. Along the Gulf of Cádiz, the boundary becomes obliquely convergent at N45∘ W (Ribeiro et al., 1996; Stich et al., 2006; Pedrera et al., 2011) with a velocity of 4.5 mm yr−1 (Nocquet and Calais, 2004; Stich et al., 2006) that accommodates intense deformation along shear zones (Terrinha et al., 2009) and through the reactivation of thrusts in the SWIM (Martínez-Loriente et al., 2013; Ramos et al., 2016). A neotectonic model across the southern plate boundary indicates that friction coefficients between 0.06 and 0.1 are the best solutions and that the maximum slip rates predicted outside the ridge occur along the Gloria Fault. Although the western segment of the Gloria Fault is almost devoid of seismicity (Jimenez-Munt and Negredo, 2003), which could indicate an expression of fault locking or slow creeping (Hensen et al., 2019), the central and eastern segments have a record of high-magnitude earthquakes, including the M8.4 event of 1941 and the M7.1 event in 1931. The deformation here is attributed to a wide zone area (Lay, 2019) or to the existence of a hydrated mantle, permitting water circulation along fractures down to 12 km below the seafloor (Batista et al., 2017).
Geodetic velocities (Garate et al., 2015; Palano et al., 2015) also reveal an important deformation in the southern sector of Iberia, while in the interior of the Iberian Peninsula the crustal deformation is locally accommodated, with rates of less than 15 nanostrain yr−1 (15 mm yr−1 per 1000 km). The stations situated in central and northern Portugal are displaced to the north around 1 mm yr−1 (Palano et al., 2015). Along the NW margin, the geodetic data provide evidence of E–W contraction, with rates of 55 nanostrain yr−1 (55 mm yr−1 per 1000 km). In the WIM, there are movements to the NW reaching 3 mm yr−1. In the active stress map of Iberia, the transition from a compressive to an extensional regime occurs around the Lisbon–Nazaré latitude (Fig. 1). A peculiarity worthy of note is the large amount of compression that exists in this area and the radical change to extension north of this transition.
Within the global picture, the present-day NNW–SSE compressional stress has been practically constant since the Miocene (Andeweg et al., 1999; de Vicente et al., 2008, and references therein) and oriented at high angles to both the northern and western coasts of Iberia, being subparallel to the tensile stresses induced by the lateral density variations along these Iberian margins (Andeweg et al., 1999).
These complex systems of forces and movements outlined above are the engines producing intraplate earthquakes in the WIM. Seismicity in the southernmost WIM is overwhelmingly clustered, as expected, on both plate boundaries, along the AGFZ with significantly complex patterns, and along the MAR. However, within mainland West Iberia and along adjacent offshore domains, away from both boundaries, seismicity has been repeatedly classified as diffuse. There is a general consensus that the magnitude and the number of events are difficult to reconcile with a typical intraplate location (Custódio et al., 2015; Krüger et al., 2020; Veludo et al., 2017; Duarte et al., 2013; Ribeiro, 2002).
The absence of ocean-bottom seismometers within the central and northern WIM, except for some local studies in neighboring areas (Krüger et al., 2020), has discouraged an in-depth study of their characteristics. In an extended study including mainland Portugal, Custódio et al. (2015) indicated that marine earthquakes collapse into well-defined clusters, while cumulative seismic moment and epicenter density decrease from south to north. The analysis of some focal mechanisms confirms that Portugal is under horizontal pressure in the NNW–SSE direction, with a great proportion of strike-slip solution and some reverse oblique mechanisms onshore (Borges et al., 2001).
The data gathered for this study belong to the permanent seismic networks of Portugal and Spain. In the Portuguese network, operated by the Instituto Português do Mar e da Atmosfera (IPMA), there is a network of 50 land stations, 5 in the Madeira archipelago and another 35 in the Azores. The Spanish network, operated by the Instituto Geográfico Nacional de España (IGN), maintains 60 stations on land and 56 in the Canary Islands. We have retrieved data from the available catalogs for January 2003 until December 2022 in the area between 38∘ and 45∘ N as well as 8∘ and 20∘ W, for earthquakes showing magnitudes >2.5.
In total, 278 events were collected from the IPMA network and 649 from the IGN network. Integrating both catalogs and selecting, in the case of coincidence, those with the lesser error in location, we compiled a final working catalog of 708 events. From these, we established several requisites of quality required to be included in this study (rms < 1, Smax < 25, Smin < 25, and Err < 75), resulting in 606 events, 352 from the Spanish network and 254 from the Portuguese network (Table 1).
Table 1Synthesis of the statistical parameters of the seismicity catalog prepared from the data of the IGN and IPMA seismic networks.
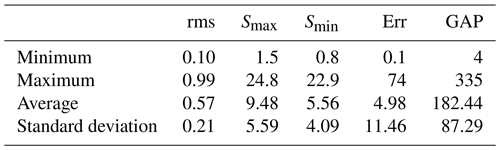
Likewise, we have included the existing historical seismicity data in the IGN catalogs and included the work of Ferrão et al. (2016), which contains information on 10 historical events that occurred within the study area. The 9 focal mechanisms considered in this work from events occurring in the marine domain have been obtained from 14 focal mechanisms included in the CMT catalog (Dziewonski et al., 1981; Ekström et al., 2012) and from the IGN Seismic Moment Tensor Database (Rueda and Mezcua, 2005).
We should be aware of some of the data limitations and reliability. The seismotectonic interpretations in continental margins under the sea present an added difficulty due to the inconclusive mapping of structures in the seafloor. Despite being well probed, the WIM contains many highs, roughness, and bathymetric heterogeneities that make it difficult to map tectonic features, specifically faults that could act as seismogenic sources. The structural variability observed along the margin added uncertainty in the data processing when introducing velocity models in the location programs of the events. Additionally, crustal depths in the margin vary, but a considerable number of events show hypocenters below the interpreted Moho depth.
The acquisition mode poses the second limitation as the land stations are located to the east, leading to a biased location error. This results in uncertainty in some locations and a lack of full azimuth to relocate with confidence. To minimize the impact of these shortcomings, only the events that comply with the requirements were considered reliable and retrieved.
- a.
Magnitude should be over >2.5.
- b.
Events should have location uncertainty of <25 km with average values <10 km and 75 km in depth. It is precisely this last parameter that poses the main problem, although the average error estimated is 4.9 km.
- c.
The average GAP (maximum angle separating two seismic stations, measured from the epicenter of an earthquake) should be <183 for all the events used in this study; the ones with higher GAP are discarded for this purpose.
The information collected was integrated through a geographic information system with (i) bathymetric information provided by the European Marine Observation and Data Network (EMODnet Bathymetry Consortium, 2020), (ii) geological structures mapped in the margin (Somoza et al., 2021), (iii) gravity and magnetic data (Granado et al., 2021; GeoMapApp – Ryan et al., 2009), and (iv) data from crustal Moho depth (Granado et al., 2021; Whitmarsh et al., 1990; GeoMapApp – Ryan et al., 2009). The ArcMap v.10.3.1 (ESRI) program was used.
3.1 Distribution, magnitudes, and focal mechanisms of the events in the WIM
Figure 2 displays the concentration of activity over the last 20 years, which extends from the coast to 19∘ W. The figure shows the distribution of events collected and processed, represented on a marine bathymetric chart (Fig. 2a), along with a density map of events (Fig. 2b) that has been calculated.
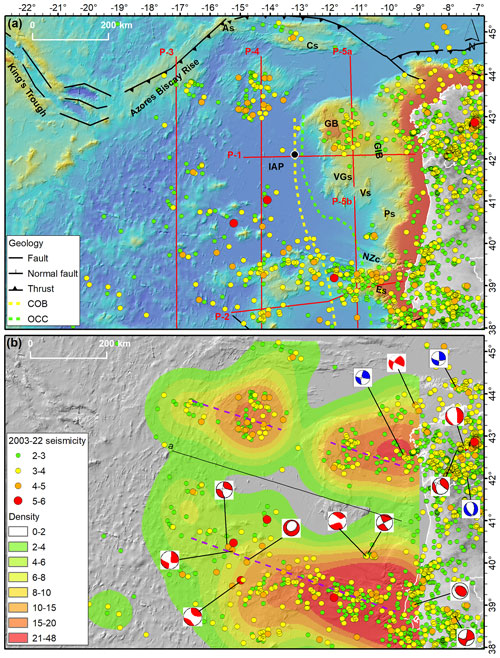
Figure 2(a) Seismicity studied in this work showing the events according to magnitude. (b) Density map of events studied in this work highlighting the alignments as purple discontinuous lines in (1) Galicia (north) and (2) Lisbon (south; see text); some focal mechanisms are shown. Seismicity catalog in the WIM in the period 2003–2022 (magnitude > 2.5, rms < 1, Smax < 25, Smin < 25, and Err < 75), according to the parameters described in Sect. 2. The topographic base is from the EMODnet Bathymetry Consortium (2020). Focal mechanisms are from the CMT (blue) catalog and IGN (red) Seismic Moment Tensor Database. P1 to P5 solid white lines show the position of the profiles in Fig. 4. The red circle is the 637 drill location cited in Boillot et al. (1988).
According to those results, events are more abundant at the coast, blending in with the onshore more dispersed seismicity. Going seawards, we start to recognize a subtle clustering, which seems to define two separated diffuse bands that extend up to 18∘ W longitude and become narrower with distance. Further towards the MAR, there are some isolated events. The orientation of these bands is about N80∘ W. Although these blurred alignments have been inferred before (Custódio et al., 2015) the discrimination of events made in this study allows removal of badly located earthquakes that add to fuzziness, resulting in a sharper image of these two seismic alignments. However, the separation into two bands is not that simple. The density maps in Fig. 2b also show that the northern band is composed of two distinct clusters that, while following the same rough NW–SE trend, are separated for more than 100 km of band space where seismicity is very scarce.
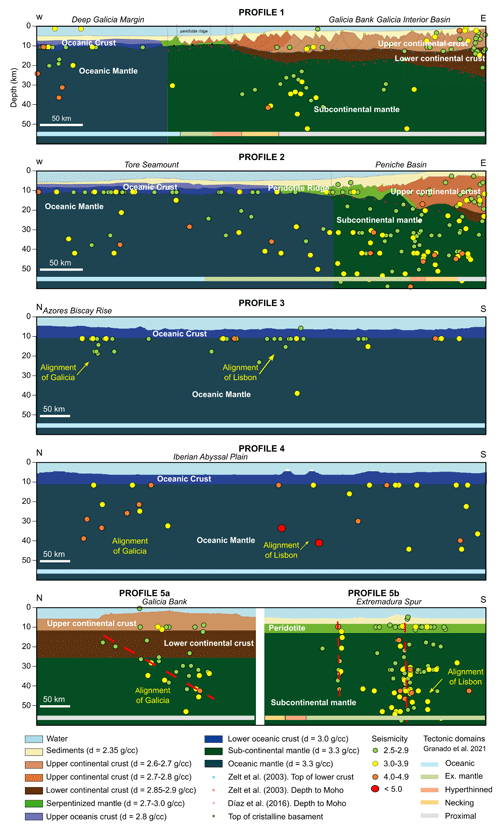
Figure 3Representation of cross-sections of seismicity at depth overlaid on crustal cross-sections modified from Amigo Marx et al. (2022), showing the crustal domains. The seismicity included in each profile comprises a 100 km band along that direction.
Figure 3 represents the depth distribution of events, superimposed on crustal cross-sections representative of the latitudes of the alignments. The bands observed are named in this study the Galicia band for the northern segment of the WIM and the Lisbon band for the central segment of the WIM. Specifically, they can be described by particular characteristics.
(a) Events offshore of Galicia, northern WIM. This density band of events is oriented N75∘ W from the coast until 17.5∘ W. It spreads over 670 km and ends in proximity to the bottom topographic expression of the Azores–Biscay rise (Fig. 2a). It seems there is clustered seismicity in two main spots along the band with a gap in the middle over 100 km of extension.
Offshore in this sector during the studied period, we registered a total of 165 events over magnitude 2.5 (eight events per year) and 21 over magnitude 4 (one event per year). Most of them are over 20 km depth and progressively shallower to the west. The depths of events include mantle depths below the geophysical inferred Moho depth, which rises from 22 km at the coast up to 11 km ocean-wards at the end of the lineation. The β value is 0.87.
In profile 1 (Fig. 3), which represents the crustal cross-section type for the Galicia Margin, there is an abundance of events in the thickest crustal areas, corresponding to the GB or to the proximal domain. The number of events is larger at the transition between the westernmost necking and hyperthinned domains interpreted by Granado et al. (2021). There is a noticeable gap in event distribution west of the Galicia Bank, followed by a second cluster in the distal margin, which coincides with an area of topographic roughness in the ocean bottom.
The figures also show that there is a non-negligible percentage of events inside the uppermost mantle, especially within the transition between the westernmost necking and hyperthinned domains. In a N–S crustal profile within the Galicia Bank cluster, a particular set of south-dipping earthquakes can be observed (profile 5a, Fig. 3). This is interpreted as the expression of the thrusts resulting from the limited Alpine convergence and incipient subduction in the Bay of Biscay that led to the partial closure of the bay (Álvarez-Marrón et al., 1997; Ayarza et al., 2004; Fernández-Viejo et al., 2012). Some of the focal mechanisms in this area have a component of a reverse fault, which would agree with this interpretation.
(b) Events offshore of Lisbon, central WIM. This second band runs for 670 km between the coast of Portugal and 16.8∘ W. We can define two subtrends, the first one oriented N80∘ W from the coast until 12.5∘ W. About 320 km away, a small bend occurs to an orientation of N75∘ E along a further 160 km until 16.8∘ W. In the central WIM, seismicity is much more abundant that in the northern WIM, although magnitudes are similar. In this sector, for the 20-year period analyzed, we recognized a total of 295 events with magnitudes over 2.5 (15 events per year), 20 with over 4–4.9 (1 yr−1), and 3 with over 5 (2 in the last decade). Most of them are concentrated in the first 30 km of depth, being progressively more superficial. The β value is 0.96.
The focal mechanisms of the events further away than 14∘ W present a significant component of strike-slip. The depth solutions reveal earthquakes nucleating below the Moho depth, which in this area decreases from 23 km at the coast to 12 km ocean-wards at the end of the lineation.
Again, in the depth distribution profiles (Fig. 3, profile 2) it seems that there are more events up to the transition between hyperextended crust and exhumed mantle and fewer events beneath the oceanic crust until the western termination of the profiles where there is an increase in the amount of seismicity and at greater depths in the oceanic domain. This decrease cannot be considered such an evident gap as in the northern WIM at the same longitudes.
There is a puzzling vertical alignment (Fig. 3, profile 5b) below the Extremadura Spur, indicating some type of structure northwards and probably a second one to the north of it. There may be a manifestation of a splay fault delimiting this topographic high. Both vertical alignments occur within the exhumed mantle section.
A detail worthy of note is that the highest-magnitude earthquakes in this area occur in the subcrustal mantle (Fig. 3, profile 4). The northernmost event is located ∼ 10 km westwards of a seamount that may be related to volcanism, as there are some volcanic edifices in the surface (Escada et al., 2022).
Although the graphics show how the depth of the earthquakes generally diminish towards the ocean along the whole WIM, there are a considerable number of earthquakes nucleating in the uppermost mantle, especially in the transition areas to exhumed mantle domain and towards the oceanic crust at the end of the alignments. This suggests that seismicity occurs in the whole margin, down to lithospheric levels. Within the central WIM, the hypocenters are slightly deeper than in the northern WIM clusters, even when crustal depths are similar in both areas. The dispersion of events is greater at around longitude 14∘ W, maybe indicating the presence of some type of seismic boundary or discontinuity in the N–S direction that traverses the whole WIM. Profiles 3 and 4 in Fig. 3 show the distribution at depth within the oceanic domains of the margin, revealing the separation of both bands. Also, they illustrate how hypocenters get shallower towards the west. In general, earthquakes along both alignments seem to cluster around marine reliefs of either tectonic origin (GB) or with volcanic additions (ES).
Earthquakes located below the Moho may indicate a strong, seismogenic upper mantle that can sustain large stresses, later released during brittle rupture. This type of “ductile earthquake” has been proposed for Wyoming lithospheric mantle (Prieto et al., 2017), and in the Newport–Inglewood fault attributed to a system of seismic asperities in ductile fault zones. Once a ductile mylonitic structure has developed a shear zone, subsequent cataclastic deformation is consistently localized in a narrow zone (Takahashi et al., 2017). The role of temperature in the rheology of the oceanic lower crust and lithospheric mantle is not well understood. Earthquakes occur throughout the oceanic crust and upper mantle, the latter with olivine-dominated rheology. The brittle–ductile transition is associated with a threshold temperature of about 600 ∘C, which represents a transition from velocity weakening to velocity strengthening, consistent with the focal depth of earthquakes in the oceanic lithosphere elsewhere (Boettcher et al., 2007).
In the area of study, but in the most southern segment of the WIM, Geissler et al. (2010) provided evidence that most of the earthquakes within the Gulf of Cádiz have hypocentral depths between 40 and 55 km. Grevemeyer et al. (2016), also in the SWIM, provide evidence for earthquakes rupturing at 30–50 km depth, hypothesizing that they are caused by proximity to the AGFZ plate boundary and by elastic behavior at the continent–ocean transition zone, which is further supported by low heat flow and the amount of regional stress caused by the Africa and Eurasia collision. Being an old passive margin, shortening and deformation of such a rigid lithosphere may cause intense deformation at mantle depths. Mantle nucleation of events may additionally be encouraged by the presence of fluids or fluid migration in the mantle, either by reducing the effective normal stress or promoting strain localization along the shear zones. The velocity gradients observed on the edges of rift domains would make them preferred sites for reactivation due to inherited strength contrasts down to mantle depths.
The focal mechanisms in the study area, corresponding to events of magnitude 4.1 to 5.9 (Table 2), indicate mostly strike-slip movement.
3.2 WIM seismicity and geophysical data
The superposition of seismicity onto different types of geophysical data can be used to infer relationships that may give hints on the seismicity sources. In the absence of confidently mapped submarine faults in the area, Fig. 4 depicts the event distribution obtained in this study overlaid on a different set of geophysical data for assessment. In the first case, the events are overlaid onto the Moho depth map. As expected, the depth of events follows crustal thickness decrease seawards. However, as many events seem to nucleate in the uppermost mantle, we must infer that the crust and the upper mantle in the WIM must be strong and that neither the Moho discontinuity nor the transition upper–lower crust is the rheological fundamental boundary concerning seismicity.
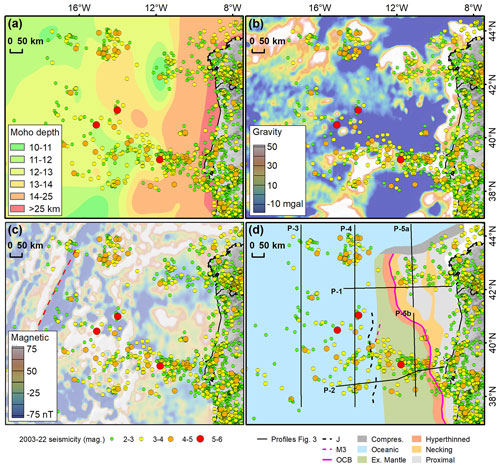
Figure 4Map of the WIM seismicity located in this study overlaid on (a) the crustal depth of the WIM (Laske et al., 2013). (b) A gravity map (Sandwell et al., 2014), (c) a magnetic map (Meyer et al., 2017), and (d) rift domains of the WIM (Granado et al., 2021).
When the seismicity is overlaid on the calculated gravity (Fig. 4b), the main relation arises from the fact that seismicity does indeed align where the basement is uplifted (i.e., Galicia Bank or Extremadura Spur) and therefore follows the gravimetric expression of thickened crustal blocks, something that could be expected given that velocity gradients have a notable influence on crustal strength. Regarding the magnetic map (Fig. 4c), it is difficult to establish a relationship due to the main N–S disposition of the oceanic magnetic anomalies. The complexity in the magnetic imprint of the continental margin reveals some relation of several events to particular magnetic structures. Finally, Fig. 4d shows the seismicity overlaid on the WIM rift domain map, which shows that seismicity does not nucleate preferentially in any domain. Although events are somewhat more abundant in the proximal domain, they do appear on exhumed mantle and hyperthinned crust, indicating that the weakness of the domains is adequate for producing earthquakes anywhere. Seismicity almost abruptly stops around the area of undisputed oceanic crust marked by anomaly M3 (Fig. 4c). Nonetheless, there are still a few events westward toward the MAR, and they follow the N80∘ W direction too. Moreover, it also shows that the central WIM seismicity happens south of the location of the change in width of the exhumed domain, therefore ignoring rift domain transitions altogether.
The concentration of seismicity at the northern and western coasts is explained in general terms as the internal deformation being the prime mode to accommodate strain induced by the convergence between Africa and Eurasia and the impossibility of propagating west of the Iberian Peninsula due to the Atlantic ridge push.
Prior to discussing the possible sources of the WIM seismicity, a summary of the characteristics of the studied events that are reliable and therefore treated as strong constraints is as follows.
- a.
Seismic events are concentrated along two distinct WNW–ESE trends parallel to the active AGFZ Eurasia–Africa plate boundary. The interval distance between bands is similar, around 300 km (Fig. 1).
- b.
Magnitude is low to moderate, and hypocentral depths include the whole crust and upper mantle.
- c.
The bands do not follow inherited tectonic trends. The events cut different rift domains and are prone to clustering along transitional areas and topographic highs. There is not an evident link to magnetic anomalies.
- d.
They do show an association with topographical highs, especially with the western border of the Galicia Bank and the northern part of the Extremadura Spur.
- e.
The few focal mechanisms available indicate overwhelmingly strike-slip types.
Based on the aforementioned observations, we review various hypotheses that have been proposed to explain seismic activity in the WIM plus a few new ideas. We can evaluate the validity of these hypotheses against the data collected over the past 2 decades.
4.1 Intraplate seismicity generated by unmapped faults – strike-slip corridors, shear zones, transform faults
Some causes for local clusters of events within the WIM have been put forward in the literature: for instance, Vázquez et al. (2008) indicated that the GB resulted from the reactivation of two major faults, forming an ample shear zone. Borges et al. (2001) related the central WIM seismicity to strike-slip movements in certain structures. Recently, Somoza et al. (2021) speculated that to the north of the Nazaré fault, seismicity responds to reactivation and inversion of thrusts situated in the ocean seamount, which even connect to faults on the continent. The Nazaré fault could be extended to the MAR, linking with the Kurchatov transform fault (Fig. 1). However, there are not enough data to support this interpretation.
In any case, local clusters do not explain the overall distribution in the WIM. We have also observed local clusters such as the southward-directed cluster in the GB related to the arrested subduction in the Bay of Biscay (Fig. 3, profile 5a) and the vertical alignment along the Extremadura Spur that may relate to either some magmatic activity or to a quasi-vertical splay fault (Fig. 3, profile 5b).
For the bigger picture and an inclusive theory for the WIM seismicity, one of the recurring hypotheses indicated by some authors was the presence of shear corridors (Custódio et al., 2015; Whitmarsh et al., 1990) due to the particular stress state of the margin and the Iberian Peninsula.
Their indifference to structures of the crust and rheological boundaries of the rifted margin supports the view that the events we are studying respond to the actual stress state, drawing an incipient silhouette of rectilinear lithospheric fractures or zones of strain parallel to the south and north of well-formed transform faults, one of them a plate boundary. These events may signal the formation of fracture zones that mimic oceanic transform faults. In contrast to the ones formed during the ocean opening, these are forming in the opposite direction from land to sea. These faults may correspond to delayed accommodation structures of the extension and opening of the Atlantic at these latitudes, where oblique extension and the jump of plate boundaries (Srivastava et al., 1990) might have impeded the formation of classic oceanic transform faults at the time.
This process would be framed within the peripatetic behaviors of the Iberian microplate. The topic of oblique spreading along the WIM has not been approached in depth. However, there is some evidence that the during opening of the Atlantic, especially during the Bay of Biscay rift, Iberia may have been “bouncing” from perpendicular to the extension direction. Favoring this option is the fact that oblique spreading is very common in slow-spreading ridges, as lithosphere is relatively cold and crustal growth is decelerated (Peyve, 2009). Another argument in agreement with this hypothesis is that mid-ocean ridges with oblique spreading are not dissected by transform faults. Shallow tectonics dictate their segmentation rather than mantle convection.
The claim for these NW–SE-oriented transform corridors to facilitate extension along the MAR, working as “pseudo-transform faults” (Fig. 5a), reinforces the need to accommodate differential stresses from south to north along the WIM, which have resulted from the rotations and subtle drift of the Iberian Plate during the past 30 Myr, together with the current NW–SE-oriented compression. In this case, the seismicity alignments may correspond to those shallow accommodation zones, similar to shear zones that act as nascent oceanic transform faults, without dissecting the ridge (yet).
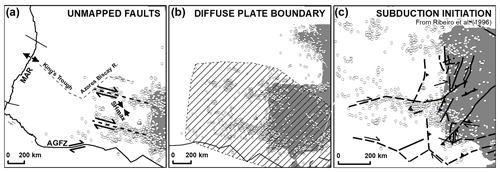
Figure 5Schematic cartoons to illustrate the different hypotheses on the origin of seismicity of the WIM. (a) Shear corridors with inverse transform faults, (b) diffuse plate boundary, and (c) initiation of subduction.
This compartmentalization of a passive margin into strike-slip narrow belts between the MAR and a deformable plate interior is more consistent with soft plate concepts than with perfectly rigid plates and narrow boundaries (Storti et al., 2007), which takes us to the next hypothesis to try to fit this seismicity into the plate tectonics theory.
4.2 A diffuse and ∼ 1000 km wide plate boundary
Plate rigidity and narrow plate boundaries are central assumptions of the original plate tectonics theory. But in the last decades evidence has been piling up to suggest that in many cases, plate boundaries can be very wide and plates may not be as rigid as assumed (Ribeiro, 2002). The diffuse plate boundary hypothesis tries to reconcile observations related to intraplate activity by proposing that large plates may be composed of a few smaller subplates and crustal blocks, so more rigid portions are in relative motion with each other separated by diffuse boundaries with clustered deformation (Gordon, 1998). The diffuse plate boundaries are much broader than traditional boundaries such as mid-ocean ridges, trenches, and/or oceanic transform faults (Zatman et al., 2000).
In this way, the passive interior of tectonic plates can be dissected by strike-slip deformation belts propagating towards MAR segments that separate adjacent, partially coupled lithospheric slivers with differential translations and deformation rates. These internal differential displacements are then accommodated by distributed deformation within the plate interior so that localized deformation is ultimately dissipated into a non-rigid plate.
Applying this model to WIM is challenging because published studies proposing soft plate deformation and the formation of weakened zones involve the spatial extension of transform faults through oceanic fracture zones, possibly weakened by serpentinization, that are used to transfer deformation from mid-ocean ridges towards neighboring plate interiors. In the WIM case, as pointed out earlier, the seismicity bands are discontinuous, do not intersect the MAR, and specifically stop before reaching the MAR, so full lithospheric detachment is not occurring. Therefore, it is more likely that these lineations may be inherited from the rifting period or from post-rift accommodation and thermal subsidence rather than the seafloor spreading stage of the Atlantic.
Along the southern Biscay margin, the Ventaniella seismicity line (Fernández-Viejo et al., 2014; López-Fernández et al., 2018) shows a similar NW–SE trend and includes earthquakes of similar magnitude, distribution, and focal mechanism as the Galicia (N) and Lisbon (S) lineation identified in the WIM. By contrast, it appears in a continental margin whose direction is perpendicular to the orientation of the WIM (the Bay of Biscay margin). If the three seismicity bands are expressions of shear corridors inside an exceptionally wide plate boundary, this hypothesis deserves credit, and the fact that they appear in two completely perpendicular-trending passive margins, even crossing oceanic and continental parts of the Iberian Plate, would support it.
Asti et al. (2022) proposed a 400 km wide, diffuse Iberia–Eurasia plate boundary transecting Iberia that would have been active during the Mesozoic and extended from the Iberian Rift system to the Armorican shelf, which was associated with the contemporaneous opening of the North Atlantic and Bay of Biscay. The deforming domain between these two boundaries was a fuzzy region, where the exact locations of different tectonic structures and rift basins are still debated. If a similar diffuse plate boundary existed today, it would be almost double in width and would encompass the entire peninsula except for its northeastern third (Pyrenees and related foreland basin).
One advantage of the diffuse plate boundary theory (Fig. 5b) is that some of the inconsistencies between the deformations inferred from plate kinematic models and geological observations (which are extraordinarily abundant in Iberian kinematics; see Barnett-Moore et al., 2016, and references therein) may be reconciled. The same amount of total displacement is partitioned between a series of subparallel strike-slip corridors, each of them accommodating a portion of the total displacement between the stable parts of the African and European plates. This would significantly broaden the range of geodynamical models in a region with such slow lithospheric deformation. If deformation between Africa and Eurasia is distributed inside Iberia, these internal corridors of deformation can accommodate the convergence of both macroplates, while synchronically assisting the E–W Atlantic extension.
Nevertheless, if a large part of Iberia belongs to a “diffuse plate boundary”, we still need to explain why the corridors are produced at that particular separation of about 300 km. Does that separation depends on the width of the subplates related to the “rigid microplate”? Does it depend on the velocity rate of the ridge? This needs further investigations out of the scope of this study, but it points to some kind of relationship to be able to form measurable strike-slip corridors between areas with contrasting crustal lithospheric thickness and plate width undergoing different stress fields. In any case, a complete radical hypothesis has been advanced in the literature, which approaches the dynamics of the WIM from a very different perspective: the closing of the Atlantic Basin.
4.3 Subduction initiation and oceanic dynamics that may be, or may have been, at play in the WIM
Several authors have suggested the possibility of a developing subduction zone in the SWIM, which could explain the moderate seismicity and the large magnitude of the 1755 Lisbon earthquake in an intraplate setting (Vilanova et al., 2003; Martínez-Loriente et al., 2021). Ribeiro et al. (1996) proposed that an incipient northward-propagating subduction zone may be nucleating at the Gorringe Bank (Fig. 5c). The ongoing terminal stage of collision between Africa and Eurasia produces compressive stresses along the SWIM, which could trigger passive margin reactivation. Gutscher et al. (2012) show evidence of subduction towards the east in the Gibraltar Arc and in the west within the southern Iberian margin NE–SW thrust systems extending 300 km along the SWIM that accommodate the convergence, while younger thrust faults are nucleating along the SWIM (Zitellini et al., 2009; Terrinha et al., 2019; Martínez-Loriente et al., 2013, 2021) and in the Tagus Abyssal Plain (Neves et al., 2009). Duarte et al. (2013) speculate that these structures may be indicative of the onset of margin tectonic inversion and the nucleation of a new subduction, which develops due to the westward propagation of the Gibraltar Arc and related subduction (Gutscher et al., 2012).
However, focusing on the central and northern WIM, away from the complexity of the plate boundary, the buoyancy of old and cold oceanic lithosphere makes the spontaneous initiation of subduction in passive margins difficult, and significant weakening mechanisms are required to fail in subduction (e.g., Stern and Gerya, 2018). The Azores, the Madeira plume, and/or the peridotite ridge in the Galicia margin may serve as potential weakening mechanisms. A 100 Myr old oceanic crust would need a significant compressive stress to fail (Zhong et al., 2019), which is not observed at the WIM.
Thermo-mechanical models suggest that magma-poor margins with hyperextended and exhumed mantle domains are favorable sites for subduction initiation, as the serpentinized mantle facilitates strain localization and the progressive development of a major shear zone that ultimately links up with a zone of high strain in the continental lower crust along the necking zone (Auzemery et al., 2021). Shearing then propagates into the mantle or initiates a subduction plate boundary. The exhumation and hydration of the mantle in the footwall of major detachments induce weak décollement layers (Brun and Beslier, 1996) prone to tectonic reactivation, which would diminish the required compressive stress to initiate subduction (Hirt and Guillot, 2013). However, the seismicity observed as we have seen it is not restricted to the exhumed mantle domains, and the focal mechanisms suggest an inverse fault. Subduction could be easier in the southern WIM as a relevant transform fault, the Gloria Fault, puts in contact two different lithospheric-age crusts, making for additional stresses and weakened lithosphere. Although the initiation of subduction by passive margin collapse is extremely unrealistic (Stern and Gerya, 2018), the westward migration and propagation of the Gibraltar Arc along the SWIM may be responsible for induced subduction initiation (Duarte et al., 2013). Although some type of underthrusting and convergence are evidenced in the south (Gutscher et al., 2012; Martínez-Loriente et al., 2021), seismicity distribution and focal mechanisms, which are predominantly strike-slip, do not support the northward propagation of a subduction interface.
4.4 Oceanic complexity, inheritance, formation of strained corridors in the Atlantic Ocean
Within the other big oceanic basin of the Earth, in the Pacific, crustal studies evidence complex tectonic settings. In triple junctions, triangular microplates without any bounding continental margins grow, as exemplified by the Galapagos microplate. Researchers have found strong evidence that, indeed, one such microplate grew to become the Pacific Plate (Boschman and van Hinsbergen, 2016). Schouten et al. (2008), studying distributed deformation at an oceanic triple junction, showed that lithospheric plates undergo significant internal deformation as their boundaries rapidly evolve, as exemplified by the Cocos–Nazca triple junction (ridge–ridge–ridge triple junction, RRR). The Cocos–Nazca rift tip does not meet the East Pacific Rise. Instead, two secondary rifts form the link. The active incipient rift is just the latest of a sequence of southeast-trending fractures that progressively stepped sideways during the last 5 Myr, successively accommodating minor N–S extension of the generated oceanic crust (Smith, 2011).
The roughness of the abyssal plain around the Kings Trough may represent the incipient and abandoned rotation of a potential mini Galapagos-like microplate. The current scars associated with the triple junction on the Kings Trough area with seafloor expression relate to inherited lithospheric fractures, which developed during the evolution of the triple point and the plate boundary migration. However, even when considering the inheritance of such lithospheric discontinuities, the seismicity in the WIM does not appear to be connected to it in particular, more than following the general NNW–SSE trend. And as Fig. 2 shows, seismicity is mainly within the continental rifted crust and not so abundant within the oceanic domains.
Palano et al. (2015) propose a current large-scale clockwise rotation that makes Iberia act as a microplate with a southern limit in the AGFZ Nubia–Eurasia convergent zone. Somoza et al. (2021) indicate that the main weakened zones along N and NW Iberia are the old inherited limits between the oceanic domains of Eurasia and Iberia (Kings Trough, Azores–Biscay rise, Jean–Charcot seamount). They question the clockwise rotation in the oceanic domain, which would be dominated by the stress derived from the ridge propagation in the segment between the Azores and Kings Trough, with a N120∘ propagation direction. They also suggest a left-lateral shear zone resulting from the northward movement of continental Iberia and the SE movement of oceanic Iberia along the OCT.
According to the possible hypothesis mentioned above, the one that presents fewer problems in this study is the idea that the pre-existing Mesozoic rift structure, together with the oblique oceanic spreading, when the plate boundary was shifting southwards towards the AGFZ, may have left weakened areas along the WIM. These inherited zones may be prone to reactivation and release seismicity under the prevailing stress regime. Inherited features revealed by the seismicity are, for instance, the old proto-subduction interface in the north of Galicia Bank (Fig. 3, profile 5) and the associated volcanic edifices around the Extremadura Spur. On the contrary, the remarkable reduction of exhumed domains towards the central parts seems to have no expression in the current seismicity distribution.
Analysis of 2 decades of seismicity data recorded by the Spanish and Portuguese seismic networks along the West Iberian passive margin (WIM), covering an area between 38 and 45∘ N latitude, shows that two NNW–SSE bands concentrate 98 % of the intraplate activity. The northern band is located offshore of Galicia, while the southern band is in offshore Lisbon. The observed seismicity is higher than expected for a passive margin and coincides with the area where the mid-Atlantic ridge lacks conspicuous transform faults. Focal mechanisms indicate strike-slip deformation, and hypocentral depths suggest crustal and upper mantle sources.
Various hypotheses have been proposed to explain this seismicity, including the formation of shear corridors and transform faults, a diffuse plate boundary, initiation of subduction, and other less understood mechanisms associated with weakened zones inherited from Mesozoic rifting, complex ocean opening, and/or triple-point migration in the Iberia microplate. Based on the parameters obtained in this study, a conservative approach to explain the WIM seismicity would be the presence of strained shear corridors that nucleate preferentially on topographical heterogeneities of the seafloor. These corridors form in response to the accommodation of two perpendicular sources of stress: the E–W extension associated with the MAR and the N–S compression from the Azores–Gibraltar fracture zone. The drastic crustal thickness variations from east to west and the nucleation of small events along multiple faults that make up the block morphology of the Mesozoic rifted margin conform the occurrence of areas prone to strain release. The presence of sub-Moho earthquakes also indicates that the upper mantle in the WIM acts as a high-strength layer, particularly beneath the hyperextended and exhumed mantle transition areas.
Several plate-scale processes may be acting simultaneously to produce the observed seismicity, such as the possible initiation of convergence-related deformation in the SWIM or the inherited compartmentalization of the lithosphere in the northern part of the margin due to triple-junction migration. Further study of seismicity in the WIM and neighboring oceanic areas, together with the arrival of seafloor stations, may provide further insights to better understand this marine intraplate clustered activity.
Seismicity data used in this study were collected from the IGN Spanish and Portuguese seismic network at https://www.ipma.pt/en/publicacoes/boletins.jsp?cmbDep=sis&idDep=sis&idTema=&curAno=-1 (IGN Spanish and Portuguese seismic network, 2022). Gravity, magnetic, and crustal data were obtained from GeoMapApp at https://www.geomapapp.org/ (Ryan et al., 2009).
GFV was responsible for the conceptualization of the work, original draft writing, and administration of the paper. CLF contributed to data curation, formal analysis, and methodologies plus figure visualization. PC contributed to formal analysis and representation. All authors participated equally in writing, editing, and reviewing the paper.
The contact author has declared that none of the authors has any competing interests.
Publisher's note: Copernicus Publications remains neutral with regard to jurisdictional claims made in the text, published maps, institutional affiliations, or any other geographical representation in this paper. While Copernicus Publications makes every effort to include appropriate place names, the final responsibility lies with the authors.
This article is part of the special issue “(D)rifting into the future: the relevance of rifts and divergent margins in the 21st century”. It is not associated with a conference.
The authors want to acknowledge the editor Tiago Alves and reviewers Sara Martínez-Loriente and Chris Elders for constructive reviews and feedback on the original submission. Chat GPT was used to improve the English language in some paragraphs on an earlier version of the paper.
This research has been supported by the Ministerio de Ciencia e Innovación (grant no. MCIU-22-PID2021-123116NB-I00) and the European Commission, Horizon 2020 (SUBIMAP (grant no. 895895)).
This paper was edited by Tiago Alves and reviewed by Sara Martínez-Loriente and Chris Elders.
Afilhado, A., Matias, L., Shiobara, H., Hirn, A., Mendes-Victor, L., and Shimamura, H.: From unthinned continent to ocean: The deep structure of the West Iberia passive continental margin at 38N, Tectonophysics, 458, 9–50, https://doi.org/10.1016/j.tecto.2008.03.002, 2008.
Álvarez-Marrón, J., Rubio, E., and Torne, M.: Subduction-related structures in the North Iberian Margin, J. Geophys. Res., 102, 22497–22511, https://doi.org/10.1029/97JB01425, 1997.
Alves, T., Moita, C., Cunha, T., Ullnaess, M., Myklebust, R., Monteiro, J. H., and Manuppella, G.: Diachronous evolution of Late Jurassic-Cretaceous continental rifting in the northwest Atlantic (west Iberian margin), Tectonics, 28, TC4003, https://doi.org/10.1029/2008TC002337, 2009.
Amigo Marx, B., Fernandez, O., Olaiz, A., Poblet, J., and Zamora, G.: On the influence of Variscan inheritance on rifting of the Western Iberian margin, Terra Nova, 34, 424–432, https://doi.org/10.1111/ter.12595, 2022.
Andeweg, B., De Vicente, G., Cloetingh, S. A. P. L., Giner, J., and Muñoz-Martin, A.: Local stress fields and intraplate deformation of Iberia: variations in spatial and temporal interplay of regional stress sources, Tectonophysics, 305, 153–164, https://doi.org/10.1016/S0040-1951(99)00004-9, 1999.
Asti, R., Saspiturry, N., and Angrand, P.: The Mesozoic Iberia-Eurasia diffuse plate boundary: A wide domain of distributed transtensional deformation progressively focusing along the North Pyrenean Zone, Earth. Sci. Rev., 230, 104040, https://doi.org/10.1016/j.earscirev.2022.104040, 2022.
Ayarza, P., Mrtinez-Catalán, J. R., Álvarez-Marrón, J., Zeyen, H., and Juhlin, C.: Geophysical constraints on the deep structure of a limited ocean-continent subduction zone at the North iberian margin, Tectonics, 23, TC101, https://doi.org/10.1029/2002TC001487, 2004.
Auzemery, A., Willingshofer, E., Sokoutis, D., Brun, J. P., and Cloetingh, S. A.: Passive margin inversion controlled by stability of the mantle lithosphere, Tectonophysics, 817, 229042, https://doi.org/10.1016/j.tecto.2021.229042, 2021.
Barnett-Moore, N., Hosseinpour, M., and Maus, S.: Assessing discrepancies between previous plate kinematic models of Mesozoic Iberia and their constraints, Tectonics, 35, 1843–1862, https://doi.org/10.1002/2015TC004019, 2016.
Batista, L., Hübscher, C., Terrinha, P., Matias, L., Afilhado, A., and Lüdmann, T.: Crustal structure of the Eurasia–Africa plate boundary across the Gloria Fault, North Atlantic Ocean, Geophys. J. Int., 209, 713–729, https://doi.org/10.1093/gji/ggx050, 2017.
Boettcher, M. S., Hirth, G., and Evans, B.: Olivine friction at the base of oceanic seismogenic zones, J. Geophys. Res., 112, B01205, https://doi.org/10.1029/2006JB004301, 2007.
Boillot, G., Girardeau, J., and Kornprobst, J.: Rifting of the Galicia margin: crustal thinning and emplacement of mantle rocks on the seafloor, in Proc. ODP, Sci. Results, 103, College Station, TX (Ocean Drilling Program), 741–756, https://doi.org/10.2973/odp.proc.sr.103.179.1988, 1988.
Borges, J. F., Fitas, A. J. S., Bezzeghoud, M., and Teves-Costa, P.: Seismotectonics of Portugal and its adjacent Atlantic Area, Tectonophysics, 337, 373–387, https://doi.org/10.1016/S0040-1951(00)00291-2, 2001.
Boschman, L. M. and van Hinsbergen, D. J. J.: On the enigmatic birth of the Pacific Plate within the Panthalassa Ocean, Sci. Adv., 2, e1600022, https://doi.org/10.1126/sciadv.1600022, 2016.
Bronner, A., Sauter, D., Manatschal, G., Péron-Pinvidic, G., and Munschy, M.: Magmatic breakup as an explanation for magnetic anomalies at magma-poor rifted margins, Nat. Geosci., 4, 549–553, https://doi.org/10.1038/ngeo1201, 2011.
Brun, J. P. and Beslier, M. O.: Mantle exhumation at passive margins, Earth. Planet. Sc. Lett., 142, 161–173, https://doi.org/10.1016/0012-821X(96)00080-5, 1996.
Buforn, E., Udías, A., and Colombás, M. A.: Seismicity, source mechanisms and seismotectonics of the Azores-Gibraltar plate boundary, Tectonophysics, 152, 89–118, 1988.
Custódio, S., Dias, N. A., Carrilho, F., Góngora, E., Rio, I., Marreiros, C., Morais, I., Alves, P., and Matias, L.: Earthquakes in western Iberia: Improving the understanding of lithospheric deformation in a slowly deforming region, Geophys. J. Int., 203, 127–145, https://doi.org/10.1093/gji/ggv285, 2015.
Dean, S. M., Minshull, T. A., Whitmarsh, R. B., and Louden, K. E.: Deep structure of the ocean-continent transition in the southern Iberia abyssal Plain from seismic refraction profiles: The IAM-9 transect at 40∘20′′ N, J. Geophys. Res., 105, 5859–5885, 2000.
De Vicente, G., Cloetingh, S., Muñoz-Martín, A., Olaiz, A., Stich, D., Vegas, R., Galindo-Zaldívar, J., and Fernández-Lozano, J.: Inversion of moment tensor focal mechanisms for active stresses around the microcontinent Iberia: Tectonic implications, Tectonics, 27, TC1009, https://doi.org/10.1029/2006TC002093, 2008.
Druet, M., Muñoz-Martín, A., Granja-Bruña, J. L., Carbó-Gorosabel, A., Llanes, P., Catalán, M., Maestro, A., Bohoyo, F., and Martín-Dávila, J.: Bouguer anomalies of the NW Iberian continental margin and the adjacent abyssal plains, J. Map. Geogr. Libr., 15, 635–641, https://doi.org/10.1080/17445647.2019.1644214, 2019.
Duarte, J. C., Rosas, F. M., Terrinha, P., Schellart, W., Boutelier, D., Gutscher, M. A., and Ribeiro, A.: Are subduction zones invading the Atlantic? Evidence form the southwest Iberia Margin, Geology, 41, 839–842, https://doi.org/10.1130/G34100.1, 2013.
Dziewonski, A. M., Chou, T. A., and Woodhouse, J. H.: Determination of earthquake source parameters from waveform data for studies of global and regional seismicity, J. Geophys. Res., 86, 2825–2852, https://doi.org/10.1029/JB086iB04p02825, 1981.
Ekström, G., Nettles, M., and Dziewonski, A. M.: The global CMT project 2004–2010: Centroid-moment tensors for 13,017 earthquakes, Phys. Earth Planet. In., 200–201, 1–9, https://doi.org/10.1016/j.pepi.2012.04.002, 2012.
EMODnet Bathymetry Consortium: EMODnet Digital Bathymetry (DTM 2020), https://doi.org/10.12770/bb6a87dd-e579-4036-abe1-e649cea9881a, 2020.
Escada, C., Represas, P., Santos, F., Pereira, R., Mata, J., and Rosas, F. M.: New evidence of Late Cretaceous magmatism on the offshore central West Iberian margin (Extremadura Spur) from potential field data, Tectonophysics, 832, 229354, https://doi.org/10.1016/j.tecto.2022.229354, 2022.
Fernandes, R. M. S., Ambrosius, B. A. C., Noomen, R., Bastos, L., Wortel, M. J. R., Spakman, W., and Govers, R.: The relative motion between Africa and Eurasia as derived from ITRF2000 and GPS data, Geophys. Res. Lett., 30, 1828, https://doi.org/10.1029/2003GL017089, 2003.
Fernández-Viejo, G., Pulgar, J. A., Gallastegui, J., and Quintana, L.: The fossil accretionary wedge of the Bay of Biscay: critical wedge analysis on depth-migrated seismic sections and geodynamical implications, J. Geol., 120, 1–18, https://doi.org/10.1086/664789, 2012.
Fernández-Viejo, G., López-Fernández, C., Domínguez-Cuesta, M. J., and Cadenas, P.: How much confidence can be conferred on tectonic maps of continental shelves? The Cantabrian-Fault case, Sci. Rep., 4, 3661, https://doi.org/10.1038/srep03661, 2014.
Ferrão, C., Bezzeghoud, M., Caldeira, B., and Borges, J. F.: The Seismicity of Portugal and Its Adjacent Atlantic Region from 1300 to 2014: Maximum Observed Intensity (MOI) Map, Seismol. Res. Lett., 87, 743–750, https://doi.org/10.1785/0220150217, 2016.
Garate, J., Martin-Davila, J., Khazaradze, G., Echeverria, A., Asensio, E., Gil, A. J., de Lacy, M. C., Armenteros, J. A., Ruiz, A. M., Gallastegui, J., Álvarez-Lobato, F., Ayala, C., Rodríguez-Caderot, G., Galindo-Zaldívar, J., Rimi, A., and Harnafi, M.: Topo-Iberia project: CGPS crustal velocity field in the Iberian Peninsula and Morocco, GPS Solut., 19, 287–295, https://doi.org/10.1007/s10291-014-0387-3, 2015.
Geissler, W. H., Matias, L., Stich, D., Carrilho, F., Jokat, W., Monna, S., Ibenbrahim, A., Mancilla, F., Gutscher, M. A., Sallares, V., and Zitellini, N.: Focal mechanisms for sub-crustal earthquakes in the Gulf of Cadiz from s dense OBS deployment, Geophys. Res. Lett., 37, L18309, https://doi.org/10.1029/2010GL044289, 2010.
Gomez de la Peña, L., Ranero, C. R., Gràcia, E., Booth-Rea, G., Azañón, J. M., Tinivella, U., and Yelles-Chaouche, A.: Evidence for a developing plate boundary in the western Mediterranean, Nat. Commun., 13, 4786, https://doi.org/10.1038/s41467-022-31895-z, 2022.
Gordon, R. G.: The plate tectonic approximation: Plate nonrigidity, diffuse plate boundaries, and global plate reconstructions, Annu. Rev. Earth Planet. Sc., 26, 615–642, 1998.
Granado, C., Muñoz-Martín, A., Olaiz, A. J., Fernández, O., and Druet, M.: 3D crustal-scale structure of the West Iberia margin: a novel approach to integrated structural characterization of passive margins, Mar. Geophys. Res., 42, 10, https://doi.org/10.1007/s11001-021-09432-2, 2021.
Grevemeyer, I., Matias, L., and Silva, S.: Mantle earthquakes beneath the South Iberia continental margin and Gulf of Cádiz – constraints from an onshore-offshore seismological network, J. Geodyn., 99, 39–50, https://doi.org/10.1016/j.jog.2016.06.001, 2016.
Grevemeyer, I., Ranero, C. R., Papenberg, C., Sallares, V., Bartolomé, R., Prada, M., Batista, L., and Neres, M.: The continent-to-ocean transition in the Iberia Abyssal Plain, Geology, 50, 615–619, 2022.
Gutscher, M. A., Dominguez, S., Westbrook, G. K., Le Roy, P., Rosas, F., Duarte, J. C., Terrinha, P., Miranda, J. M., Graindorge, D., Gailler, A., Sallares, V., and Bartolome R.: The Gibraltar subduction: a decade of new geophysical data, Tectonophysics, 574, 72–91, https://doi.org/10.1016/j.tecto.2012.08.038, 2012.
Hensen, C., Duarte, J. C., Vannucchi, P., Mazzini, A., Lever, M. A., Terrinha, P., Géli, L., Henry, P., Villinger, H., Morgan, J., Schmidt, M., Gutscher, M.-A., Bartolome, R., Tomonaga, Y., Polonia, A., Gràcia, E., Tinivella, U., Lupi, M., Çağatay, M. N., Elvert, M., Sakellariou, D., Matias, L., Kipfer, R., Karageorgis, A. P., Ruffine, L., Liebetrau, V., Pierre, C., Schmidt, C., Batista, L., Gasperini, L., Burwicz, E., Neres, M., and Nuzzo, M.: Marine Transform Faults and Fracture Zones: A Joint Perspective Integrating Seismicity, Fluid Flow and Life, Front. Earth Sci., 7, 39, https://doi.org/10.3389/feart.2019.00039, 2019.
Hirt, G. and Guillot, S.: Rheology and tectonic significance of serpentine, Elements, 9, 107–113, https://doi.org/10.2113/gselements.9.2.107, 2013.
IGN Spanish and Portuguese seismic network: Seismicity data, IPMA [data set], https://www.ipma.pt/en/publicacoes/boletins.jsp?cmbDep=sis&idDep=sis&idTema=&curAno=-1, last access: December 2022.
Jimenez-Munt, I. and Negredo, A.: Neotectonic modeling of the western part of the Africa-Eurasia plate boundary: from the Mid-atlantic ridge to Algeria, Earth Planet. Sc. Lett., 205, 257–271, https://doi.org/10.1016/S0012-821X(02)01045-2, 2003.
King, M. T. and Welford, J. K.: Advances in deformable plate tectonic models: 2. Reconstructing the southern North Atlantic back through time, Geochem. Geophy. Geosy., 23, e2022GC010373, https://doi.org/10.1029/2022GC010373, 2022.
King, M. T., Welford, J. K., and Peace, A. L.: Investigating the role of the Galicia Bank on the formation of the North West Iberian margin using deformable plate tectonic models, Tectonophysics, 789, 228537, https://doi.org/10.1016/j.tecto.2020.228537, 2020.
Krüger, F., Dahm, T., and Hannemann, K.: Mapping of Eastern North Atlantic Ocean seismicity from Po/So observations at a mid-aperture seismological broad-band deep sea array, Geophys. J. Int., 221, 1055–1080, https://doi.org/10.1093/gji/ggaa054, 2020.
Laske, G., Masters, G., Ma, Z., and Pasyanos, M.: Update on CRUST1.0 – A 1-degree Global Model of Earth's Crust, Geophys. Res. Abstr., EGU2013-2658, EGU General Assembly 2013, Vienna, Austria, 2013.
Lay, T.: Reactivation of Oceanic Fracture Zones in Large Intraplate Earthquakes?, in: Transform Plate Boundaries and Fracture Zones, edited by: Duarte, J. C., Elsevier, 89–104, https://doi.org/10.1016/B978-0-12-812064-4.00004-9, 2019.
López-Fernández, C., Fernández-Viejo, G., Olona, J., and Llana-Fúnez, S.: Intraplate seismicity in Northwest Iberia along the trace of the Ventaniella fault: a case for fault intersection at depth, B. Seismol. Soc. Am., 108, 604–618, https://doi.org/10.1785/0120170215, 2018.
Lymer, G. and Reston, T. J.: The West Iberian margin: past and current research concepts and future challenges. Continental rifted margins 2. Case examples, edited by: Peron-Pinvidic, G., Wiley and Sons, 256 pp., https://doi.org/10.1002/9781119986959.ch1, 2022.
Macchiavelli, C., Vergés, J., Schettino, A., Fernàndez, M., Turco, E., Casciello, E., Torne, M., Pierantoni, P. P., and Tunini, L.: A new southern North Atlantic isochron map: Insights into the drift of the Iberian Peninsula since the Late Cretaceous, J. Geophys. Res., 122, 9603–9626, https://doi.org/10.1002/2017JB014769, 2017.
Malod, J. A. and Mauffret, M.: Iberian plate motions during the Mesozoic, Tectonophysics, Geol. Soc. Lond., 184, 261–278, 1990.
Martínez-Loriente, S., Gracia, E., Bartolome, R., Sallarès, V., Connors, C., Perea, H., Lo Iacono, C., Klaeschen, D., Terrinha, P., Danobeitia, J. J., and Zitellini, N.: Active deformation in old oceanic lithosphere and significance for earthquake hazard: Seismic imaging of the Coral Patch Ridge area and neighboring abyssal plains (SW Iberian Margin), Geochem. Geophys. Geosy., 14, 2206–2231, 2013.
Martínez-Loriente, S., Sallares, V., and Gracia, E.: The Horseshoe abyssal plain thrust could be the source of the 1755 Lisbon earthquake and tsunami, Commun. Earth. Environ., 2, 145, https://doi.org/10.1038/s43247-021-00216-5, 2021.
Merino, I., Ranero, C. R., Prada, M., Sallares, V., and Grevemeyer, I.: The Rift and Continent-Ocean Transition Structure under the Tagus Abyssal Plain West of the Iberia, J. Geophys. Res.-Sol. Ea., 126, e2021JB022629, https://doi.org/10.1029/2021JB022629, 2021.
Meyer, B., Saltus, R., and Chulliat, A.: EMAG2v3: Earth Magnetic Anomaly Grid (2-arc-minute resolution), Version 3, NOAA National Centers for Environmental Information, https://doi.org/10.7289/V5H70CVX, 2017.
Morel, J. L. and Meghraoui, M.: Goringe-Alboran-Tell Tectonic Zone: A Transpression System along the Africa-Eurasia Plate Boundary, Geology, 24, 755–758, https://doi.org/10.1130/0091-7613(1996)024<0755:GATTZA>2.3.CO;2, 1996.
Murillas, J., Mougenot, D., Boulot, G., Comas, M. C., Banda, E., and Mauffret, A.: Structure and evolution of the Galicia Interior Basin (Atlantic western Iberian continental margin), Tectonophysics, 184, 297–319, https://doi.org/10.1016/0040-1951(90)90445-E, 1990.
Neves, M. C., Terrinha, P., Alfilhado, A., Moulin, M., Matias, L., Rosas, F.: Response of a muti-domain continetal margin to compression: Study from seismic reflection-refraction and numerical modelling in the Tagus Abyssal plain, Tectonophysics, 468, 113–130, https://doi.org/10.1016/j.tecto.2008.05.008, 2009.
Nirrengarten, M., Manatschal, G., Tugend, J., Kusznir, N. J., and Sauter, D.: Nature and origin of the J-magnetic anomaly offshore Iberia-Newfoundland: implications for plate reconstructions, Terra Nova, 29, 20–28, https://doi.org/10.1111/ter.12240, 2017.
Nirrengarten, M., Manatschal, G., Tugend, J., Kusznir, N. J., and Sauter, D.: Kinematic Evolution of the Southern North Atlantic: Implications for the Formation of Hyperextended Rift Systems, Tectonics, 37, 89–118, https://doi.org/10.1002/2017TC004495, 2018.
Nocquet, J. M.: Present-day kinematics of the Mediterranean: A comprehensive overview of GPS results, Tectonophysics, 579, 220–242, https://doi.org/10.1016/j.tecto.2012.03.037, 2012.
Nocquet, J. M. and Calais, E.: Geodetic measurements of crustal deformation in the western Mediterranean and Europe, Pure Appl. Geophys., 161, 661–681, https://doi.org/10.1007/s00024-003-2468-z, 2004.
Palano, M., Gonzalez, P. J., and Fernandez, J.: The diffuse plate boundary of Nubia and Iberia in the western Mediterranean: crustal deformation evidence for viscous coupling and fragmented lithosphere, Earth Planet. Sc. Lett., 430, 439–447, https://doi.org/10.1016/j.epsl.2015.08.040, 2015.
Peace, A. L., Welford, J. K., Ball, P. J., and Nirrengarten, M.: Deformable plate tectonic models of the southern North Atlantic, J. Geodyn., 128, 11–37, https://doi.org/10.1016/j.jog.2019.05.005, 2019.
Pedrera, A., Ruiz-Constán, A., Galindo-Zaldívar, J., Chalouan, A., Sanz de Galdeano, C., Marín-echado, C., Ruano, P., Benmakhlouf, M. Akil, M., López-Garrido, A. C., Chabli, A., Ahmamou, M., and González-Castillo, L.: Is there an active subduction beneath the Gibraltar orogenic arc. Constraints from Pliocene to Present-day stress field, J. Geodyn., 52, 83–96, https://doi.org/10.1016/j.jog.2010.12.003, 2011.
Pedrera, A., García-Senz, J., Ayala, C., Ruiz-Constán, A., Rodriguez-Fernández, L. R., Robador, A., and González Menéndez, L.: Reconstruction of the exhumed mantle across the North Iberian Margin by crustal-scale 3-D gravity inversion and geological cross section, Tectonics, 413, 150–154, https://doi.org/10.1002/2017TC004716, 2017.
Péron-Pinvidic, G. and Manatschal, G.: The final rifting evolution at deep magma-poor passive margins from Iberia-Newfoundland: a new point of view, Int. J. Earth Sci., 98, 1581–1597, https://doi.org/10.1007/s00531-008-0337-9, 2009.
Péron-Pinvidic, G., Manatschal, G., Minshull, T. A., and Sawyer, D. S.: Tectonosedimentary evolution of the deep Iberia-Newfoundland margins: evidence for a complex breakup history, Tectonics, 26, TC2011, https://doi.org/10.1029/2006TC001970, 2007.
Pereira, R., Alves, T., and Cartwright, J.: Post rift compression of the SW Iberian margin (eastern North Atlantic): a case for prolonged inversion in the ocean-continent transition zone, J. Geol. Soc., 168, 1249–1263, https://doi.org/10.1144/0016-76492010-151, 2011.
Pereira, R., Alves, T., and Mata, J.: Alternating crustal architecture in West Iberia: a review of its significance in the context of NE Atlantic rifting, J. Geol. Soc., 174, 522–540, https://doi.org/10.1144/jgs2016-050, 2016.
Peyve, A. A.: Accretion of oceanic crust under conditions of oblique spreading, Geotectonics, 43, 87–99, https://doi.org/10.1134/S0016852109020022, 2009.
Pinheiro, L. M., Whitmarsh, R. B., and Miles, P. R.: The ocean-continent boundary off the western continental margin of Iberia-II. Crustal structure in the Tagus Abyssal Plain, Geophys. J. Int., 109, 106–124, https://doi.org/10.1111/j.1365-246X.1992.tb00082.x, 1992.
Pinheiro, L. M., Wilson, R. C. L., Pena dos Reis, R., Whitmarsh, R. B., and Ribeiro, A.: The Western Iberian Margin: geophysical and geological overview, in: Proc. ODP, Sci. Results, 149, edited by: Whitmarsh, R. B., Sawyer, D. S., Klaus, A., and Masson, D. G., College Station, TX (Ocean Drilling Program), 3–23, https://doi.org/10.2973/odp.proc.sr.149.246.1996, 1996.
Prieto, G. A., Froment, B., Yu, C., Poli, P., and Abercrombie, R.: Earthquake rupture below the brittle-ductile transition in continental lithospheric mantle, Sci. Adv., 3, e1602642, https://doi.org/10.1126/sciadv.1602642, 2017.
Ramos, A., Fernández, O., Terrinha, P., and Muñoz, J. A.: Extension and inversion structures in the Tethys-Atlantic linkage zone, Algarve Basin, Portugal, Int. J. Earth Sci., 105, 1663–1679, https://doi.org/10.1007/s00531-015-1280-1, 2016.
Ribeiro, A.: Soft Plate and Impact Tectonics, Springer Verlag, Heidelberg, 1–324, https://doi.org/10.1007/978-3-642-56396-6, 2002.
Ribeiro, A., Cabral, J., Baptista, R., and Matias, L.: Stress pattern in Portugal mainland and the adjacent Atlantic region, West Iberia, Tectonics, 15, 641–659, https://doi.org/10.1029/95TC03683, 1996.
Roest, W. R. and Srivastava, S. P.: Kinematics of the plate boundaries between Eurasia, Iberia and Africa in the North-Atlantic from the Late Cretaceous to the present, Geology, 19, 613–616, https://doi.org/10.1130/0091-7613(1991)019<0613:KOTPBB>2.3.CO;2, 1991.
Rueda, J. and Mezcua, J.: Near-real-time Seismic Moment-tensor Determination in Spain, Seismol. Res. Lett., 276, 455–465, https://doi.org/10.1785/gssrl.76.4.455, 2005.
Ryan, W. B. F., Carbotte, S. M., Coplan, J., O'Hara, S., Melkonian, A., Arko, R., Weissel, R. A., Ferrini, V., Goodwillie, A., Nitsche, F., Bonczkowski, J., and Zemsky, R.: Global Multi-Resolution Topography (GMRT) synthesis data set, Geochem. Geophys. Geosy., 10, Q03014, https://doi.org/10.1029/2008GC002332, 2009 (data available at: https://www.geomapapp.org/, last access: January 2023).
Sallarès, V., Martínez-Loriente, S., Prada, M., Gràcia, E., Ranero, C., Gutscher, M. A., Bartolome, R., Gailler, A., Dañobeitia, J. J., and Zitellini, N.: Seismic evidence of exhumed mantle rock basement at the Gorringe Bank and the adjacent Horseshoe and Tagus abyssal plains (SW Iberia), Earth. Planet. Sc., 365, 120–131, https://doi.org/10.1016/j.epsl.2013.01.021, 2013.
Sandwell, D. T., Müller, R. D., Smith, W. H. F., Garcia, E., and Francis, R.: New global marine gravity model from CryoSat-2 and Jason-1 reveals buried tectonic structure, Science, 346, 65–67, https://doi.org/10.1126/science.1258213, 2014.
Schouten, H., Smith, D. K., Montési, L. G. J., Zhu, W., and Klein, E. M.: Cracking of lithosphere north of the Galapagos triple junction, Geology, 36, 339–342, https://doi.org/10.1130/G24431A.1, 2008.
Schulmann, K., Edel, J. B., Martínez Catalán, J. R., Mazur, S., Guy, A., Lardeaux, J. M., Ayarza, P., and Palomeras, I.: Tectonic evolution and global crustal architecture of the European Variscan belt constrained by geophysical data, Earth. Sci. Rev., 234, 104195, https://doi.org/10.1016/j.earscirev.2022.104195, 2022.
Serpelloni, E., Vannucci, G., Pondrelli, S., Argnani, A., Casula, G., Anzidei, M., Baldi, P., and Gasperini, P.: Kinematics of the Western Africa-Eurasia plate boundary from focal mechanisms and GPS data, Geophys. J. Int., 169, 1180–1200, https://doi.org/10.1111/j.1365-246X.2007.03367.x, 2004.
Sibuet, J., Srivastava, S. P., and Spakman, W.: Pyrenean orogeny and plate kinematics, J. Geophys. Res., 109, B08104, https://doi.org/10.1029/2003JB002514, 2004.
Smith, D. K.: Grid(s) of multibeam bathymetry at Galapagos Triple Junction, Palisades, New York, Integr. Earth Data Appl., https://doi.org/10.1594/IEDA/100005, 2011.
Srivastava, S. P. and Roest, W. R.: King's Trough: reactivated pseudo-fault of a propagating rift, Geophys. J. Int., 108, 143–150, https://doi.org/10.1111/j.1365-246X.1992.tb00845.x, 1992.
Srivastava, S. P., Schouten, H., Roest, W. R., Klitgord, K., Kovacs, L. C., Verhoef, J., and Macnab, R.: Iberian plate kinematics: a jumping plate boundary between Eurasia and Africa, Nature, 344, 756–759, 1990.
Stich, D., Serpelloni, E., Mancilla, F. L., and Morales, J.: Kinematics of the Iberia-Maghreb plate contact from seismic moment tensors and GPS observations, Tectonophysics, 426, 295–317, https://doi.org/10.1016/j.tecto.2006.08.004, 2006.
Soares, D. M., Alves, T. A., and Terrinha, P.: The breakup sequence and associated lithospheric breakup surface: Their significance in the context of rifted continental margins (West Iberia and Newfoundland margins, North Atlantic), Earth. Planet. Sc. Lett., 355–356, 311–326, 2012.
Somoza, L., Medialdea, T., González, F. J., León, R., Palomino, D., Rengel, J., Fernández-Salas, L. M., and Vázquez, J.: Morphostructure of the Galicia continental Margin and Adjacent Deep Ocean Floor: From Hyperextended Rifted to Convergent Margin Styles, Mar. Geol., 407, 299–315, https://doi.org/10.1016/j.margeo.2018.11.011, 2019.
Somoza, L., Medialdea, T., González, F. J., Machancoses, S., Candón, J. A., Cid, C., Calado, A., Afonso, A., Pinto Ribeiro, L., Blasco, I., Albuquerque, M., Asensio-Ramos, M., Bettencourt, R., De Ignacio, C., López-Pamo, E., Ramos, B., Rincón-Tomás, B., Santofimia, E., Souto, M., Tojeira, I., Viegas, C., and Madureira, P.: High-Resolution Multibeam Bathymetry of the Northern Mid-Atlantic Ridge at 45–46∘ N: the Moytirra Hydrothermal Field, J. Maps, 17, 184–196, https://doi.org/10.1080/17445647.2021.1898485, 2021.
Stern, R. J. and Gerya, T.: Subduction initiation in nature and models: A review, Tectonophysics, 746, 173–198, https://doi.org/10.1016/j.tecto.2017.10.014, 2018.
Storti, F., Soto Marin, R., Rossetti, R., and Casas Sainz, A. M.: Evolution of experimental thrust wedges accreted from along-strike tapered, silicone-floored multilayers, J. Geol. Soc., 164, 73–85, https://doi.org/10.1144/0016-76492005-186, 2007.
Takahashi, M., van den Ende, M. P. A., Niemeijer, A. R., and Spiers, C. J.: Shear localization in a mature mylonitic rock analog during fast slip, Geochem. Geophy. Geosy., 18, 513–530, https://doi.org/10.1002/2016GC006687, 2017.
Terrinha, P., Matias, L., Vicente, J., Duarte, J., Luís, J., Pinheiro, L., Lourenço, N., Diez, S., Rosas, F., Magalhães, V., Valadares, V., Zitellini, N., Roque, C., and Mendes Víctor, L.: Morphotectonics and Strain Partitioning at the Iberia-Africa Plate Boundary from Multibeam and Seismic Reflection Data, Mar. Geol., 267, 156–174, https://doi.org/10.1016/j.margeo.2009.09.012, 2009.
Terrinha, P., Ramos, A., Neres, M., Valadares, V., Duarte, J., Martínez-Loriente, S., Silva, S., Mata, J., Kullberg, J. C., Casas-Sainz, A., Matias, L., Fernández, O., Muñoz, J. A., Ribeiro, C., Font, E., Neves, C., Roque, C., Rosas, F., Pinheiro, L., Bartolomé, R., Sallarès, V., Magalhães, V., Medialdea, T., Somoza, L., Gràcia, E., Hensen,C., Marc-André Gutscher, M. A., Ribeiro, A., and Zitellini, N.: The alpine orogeny in the West and Southwest Iberia margins, in: The Geology of Iberia: A Geodynamic Approach, Regional Geology Reviews, edited by: Quesada, C. and Oliveira, J., Springer, Cham., 487–505, https://doi.org/10.1007/978-3-030-11295-0_11, 2019.
Tucholke, B. E., Sawyer, D., and Sibuet, J. C.: Breakup of the Newfoundland-Iberia rift, in: Imaging, Mapping and Modelling Continental Lithosphere Extension and Breakup, edited by: Karner, G. D., Manatschal, G., and Pinheiro, L. M., Geol. Soc. Lond. Spec. Publ., 282, 9–46, https://doi.org/10.1144/sp282.2, 2007.
Vázquez, J. T., Medialdea, T., Ercilla, G., Somoza, L., Estrada, F., Fernández-Puga, M. C., Gallart, J., Gràcia, E., Maestro, A., and Sayago, M.: Cenozoic deformational structures on the Galicia Bank Region (NW Iberian continental margin), Mar. Geol., 249, 128–149, 2008.
Veludo, I., Dias, N. A., Fonseca, P. E., Matias, L., Carrilho, F., Haberland, C., and Villaseñor, A.: Crustal seismic structure beneath Portugal and southern Galicia (Western Iberia) and the role of Variscan inheritance, Tectonophysics, 717, 645–664, https://doi.org/10.1016/j.tecto.2017.08.018, 2017.
Vilanova, S. P., Nunes, C. F., and Fonseca, J. F. B. D.: Lisbon 1755: A Case of Triggered Onshore Rupture?, B. Seismol. Soc. Am., 93, 2056–2068, https://doi.org/10.1785/0120020245, 2003.
Vissers, R. and Meijer, P. T.: Mesozoic rotation of Iberia: Subduction in the Pyrenees?, Earth Sci. Rev., 110, 93–110, https://doi.org/10.1016/j.earscirev.2011.11.001, 2012.
Whitmarsh, R. B., Miles, P. R., and Mauffret, A.: The ocean-continent boundary off the western continental margin of Iberia-I. Crustal structure at 40∘30′ N, Geophys. J. Int., 103, 509–531, https://doi.org/10.1111/j.1365-246X.1990.tb01788.x, 1990.
Whitmarsh, R. B., Pinheiro, L. M., Miles, P. R., Recq, M., and Sibuet, J. C.: Thin crust at the Western Iberia Ocean-Continent Transition and ophiolites, Tectonics, 12, 1230–1239, https://doi.org/10.1029/93TC00059, 1993.
Zatman, S.: On steady rate coupling between an elastic upper crust and a viscous interior, Geophys. Res. Lett., 27, 2421–2424, https://doi.org/10.1029/2000GL011592, 2000.
Zitellini, N., Gracia, E., Matias, L., Terrinha, P., Abreu, M. A., Dealteris, G., Henriet, J. P., Dañobeitia, J. J., Masson, D. G., Mulder, T., Ramella, R., Somoza, L., and Diez, S.: The quest for the Africa-Eurasia plate boundary west of the Strait of Gibraltar, Earth Planet. Sc. Lett., 280, 13–50, https://doi.org/10.1016/j.epsl.2008.12.005, 2009.
Zhong, T., Gordon, R. G., and Wang, C.: Oblique seafloor spreading across intermediate and superfast spreading centers, Earth Planet. Sc. Lett., 495, 146–156, https://doi.org/10.1016/j.epsl.2018.05.001, 2019.
- Abstract
- Introduction
- Gathering seismicity in the WIM
- Results, clusters, and trends
- Causes of the intraplate seismicity along the West Iberian Margin
- Conclusions
- Data availability
- Author contributions
- Competing interests
- Disclaimer
- Special issue statement
- Acknowledgements
- Financial support
- Review statement
- References
- Abstract
- Introduction
- Gathering seismicity in the WIM
- Results, clusters, and trends
- Causes of the intraplate seismicity along the West Iberian Margin
- Conclusions
- Data availability
- Author contributions
- Competing interests
- Disclaimer
- Special issue statement
- Acknowledgements
- Financial support
- Review statement
- References