the Creative Commons Attribution 4.0 License.
the Creative Commons Attribution 4.0 License.
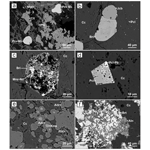
Rare Earth element distribution on the Fuerteventura Basal Complex (Canary Islands, Spain): a geochemical and mineralogical approach
Inmaculada Menéndez
Luis Quevedo
Jorge Yepes
Ramón Casillas
Agustina Ahijado
Jorge Méndez-Ramos
José Mangas
The Fuerteventura Basal Complex comprises Oligocene and Miocene ultra-alkaline-carbonatitic magmatic pulses with outcrops that extend across kilometer-scale areas in some specific sectors of this oceanic island. Additionally, there is evidence of associated weathering materials that affect these magmatic lithologies. These alkaline magmatic rocks (including trachytes, phonolites, syenites, melteigites, and ijolites), carbonatites, and their associated weathering products underwent a preliminary evaluation of rare Earth element (REE) contents based on mineralogical and geochemical studies. REE concentrations in carbonatites of about 10 300 ppm REY (REEs plus yttrium) have been detected in volumes comparable to other locations hosting significant deposits of these critical elements worldwide. Conversely, alkaline magmatic rocks and the resulting weathering products display limited REE contents. Notably, REEs in carbonatites are associated with primary accessory phases such as REE-bearing pyrochlore and britholite and secondary monazite. The results obtained in the carbonatites of Fuerteventura underscore the interest in studying the concentrations of critical elements, such as REEs, within a non-conventional geological setting like oceanic islands. However, due to intricate structural attributes, the irregular distribution of these mineralizations, and possible land use and environmental constraints, additional future detailed investigations are imperative to ascertain the real potential of these REE concentrations.
- Article
(17469 KB) - Full-text XML
-
Supplement
(318 KB) - BibTeX
- EndNote
The European Commission (EC) is spearheading efforts to combat climate change through the European Green Deal (EGD) with the goal of achieving a carbon-neutral continent by 2050 (European Commission, 2019). This initiative entails transitioning to green technologies which heavily rely on rare Earth elements (REEs) for applications like renewable energy systems and electric vehicles (Acosta-Mora et al., 2018; Alonso et al., 2012; Chakhmouradian and Wall, 2012; Massari and Ruberti, 2013; Méndez-Ramos et al., 2013; Wondraczeck et al., 2015).
According to the International Union of Pure and Applied Chemistry (IUPAC), REEs comprise a group of 17 chemical elements: scandium (Sc), yttrium (Y), and the 15 members of the lanthanide series (Connelly et al., 2005). The term “rare” is confusing because, even though REEs seldom occur in pure mineral phases, their average concentration in the Earth's crust is around 125 ppm, surpassing other metals such as copper, gold, or platinum (Long et al., 2010; Rudnick and Gao, 2014).
Given their pivotal role in modern industry and green technologies, as well as the projected increase in demand for REEs in the coming years, governments worldwide are actively promoting the advancement of knowledge regarding REE distribution in the geological environment (Barteková and Kemp, 2016; European Commission, 2023a, b).
The study of REEs has primarily centered on investigating non-conventional HREE (heavy rare Earth element) geological settings such as soils and weathering products (Braun et al., 1993; Q. Wang et al., 2010; X. Wang et al., 2013; Berger et al., 2014; Aiglsperger et al., 2016; Torró et al., 2017; Reinhardt et al., 2018; Borst et al., 2020) but also traditional and well-known LREE (light rare Earth element)-bearing lithologies, such as carbonatites (Goodenough et al., 2016; Yang et al., 2019; Pirajno and Yu, 2022).
Carbonatites are igneous rocks formed by carbonate mantle melts and are genetically associated with a wide range of mafic, ultramafic, and alkaline silicate rocks (Yaxley et al., 2022). Although carbonates such as calcite or dolomite are their main forming minerals, a significant portion of carbonatites contain accessory phases enriched in critical metals such as REEs (Christy et al., 2021). REEs can be contained in fluorcarbonates (e.g., bastnäsite, parisite, huanghoite, and synchysite), phosphates (e.g., monazite and rhabdophane), silicates (e.g., allanite), or even oxides (e.g., REE-bearing pyrochlore and cerianite). These accessory minerals make carbonatites the main current REE source, representing 86.5 % of the deposits under exploitation for these elements (Liu et al., 2018). However, although carbonatites are rare rocks predominantly found in continental rifts associated with cratons (Humphreys-Williams et al., 2021), they have exceptionally been described in other geological contexts, most notably oceanic islands associated with hotspots, such as Cabo Verde (Mourão et al., 2010; De Ignacio et al., 2012) or Fuerteventura in the Canary Islands (Mangas et al., 1994; Carnevale et al., 2021).
The petrogenesis of carbonatites is still a debated topic (Anenburg et al., 2021; Yaxley et al., 2022). Different processes have been proposed for their formation, although there is a consensus that they originate from primary fusion processes derived from a carbonated mantle (Kamenetsky et al., 2021). For the specific case of the oceanic carbonatites, this debate is even more lively. Doucelance et al. (2010) suggested a shallow origin from low-degree partial melting at the base of the oceanic lithosphere. Other authors have proposed the involvement of an unmixing process linking to alkaline magma suites (Weidendorfer et al., 2016), the action of hydrothermal fluids of marine origin enriched in Ca that would have serpentinized the mantle (Park and Rye, 2013), or even the contribution of recycled marine carbonates through subduction or assimilated in shallow magma chambers (Démeny et al., 1998; Hoernle et al., 2002; Doucelance et al., 2014).
The present study focuses on the mineralogical and geochemical analysis of carbonatites and associated alkaline igneous rocks, as well as their weathering products, in three distinct sectors in the western region of Fuerteventura (Canary Islands, Spain; see Fig. 1). The primary objective of this research is to deepen our understanding of REE distribution in these materials within the exotic geological context of an oceanic island associated with intraplate magmatism.
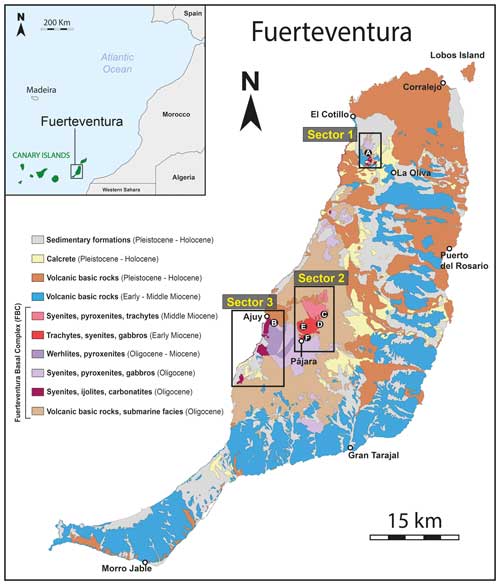
Figure 1Simplified geological map of Fuerteventura island (modified from Balcells et al., 1994) showing the location of the three study sectors for the assessment of REE content in the FBC. Additionally, the studied weathering profiles are also indicated as (A) Agua Salada ravine, (B) Aulagar ravine, (C) Palomares ravine, (D) FV-30 road, (E) Las Peñitas quarry, and (F) Pájara.
2.1 The Canary Island Seamount Province
The Canary Islands archipelago, located between 27 and 30° N latitude, is part of the Canary Island Seamount Province (CISP). This volcanic region forms a band of approximately 1300 km in length and 350 km in width, running parallel to the African continental margin. Within the CISP, there are over 100 seamounts and up to 8 emerged islands, namely El Hierro, La Palma, La Gomera, Tenerife, Gran Canaria, Lanzarote, Fuerteventura, and the Savage islands (Courtillot et al., 2003; Schmincke and Sumita, 2010; van den Bogaard, 2013). Based on magnetic anomaly measurements and dating of both emerged and submarine igneous materials, volcanic activity in the CISP spans more than 142 Ma, from the Early Cretaceous to the present day (Frisch, 2012; van den Bogaard, 2013; Longpré and Felpeto, 2021).
2.2 Fuerteventura island
Fuerteventura, the easternmost island of the Canarian archipelago, along with Lanzarote, forms the emergent crest of the eastern Canarian volcanic ridge which is located approximately 100 km offshore from the Moroccan coast (Fig. 1). Fuerteventura is the oldest island in the archipelago, with its initial stages of formation linked with submarine volcanic activity, dating to the Oligocene (∼ 34 Ma). The first episodes of subaerial volcanism occurred around ∼ 23 Ma (Coello et al., 1992; Ancochea et al., 1996; Pérez-Torrado et al., 2023).
Fuerteventura is characterized by the occurrence of three distinct main geological units arranged in order from oldest to youngest: the Fuerteventura Basal Complex (FBC), the Miocene subaerial volcanic units, and the Pliocene–Quaternary volcano sedimentary facies (Fúster et al., 1968; Le Bas et al., 1986; Muñoz et al., 2005; Gutiérrez et al., 2006; Troll and Carracedo, 2016).
2.2.1 The Fuerteventura Basal Complex
The FBC unit mainly outcrops in the western part of the island (Fig. 1). Two different groups of lithofacies may be distinguished: (1) Early Jurassic to Late Cretaceous oceanic crust materials (Steiner et al., 1998) constituted by mid-ocean ridge basalts and oceanic sediments and (2) Oligocene submarine and transitional volcanic rocks associated with plutonic bodies and dike swarms (Feraud et al., 1985; Hobson et al., 1998; Gutiérrez et al., 2006). In this second group, a set of lithologies can be distinguished related to an ultra-alkaline-carbonatitic magmatic pulse that occurred ∼ 25 Ma (Le Bas, 1981; Barrera et al., 1981; Balogh et al., 1999). Additionally, alkaline ultramafic, mafic, and felsic plutonic rocks such as wehrlites, pyroxenites, gabbros, and syenites intruded the previously existing Oligocene materials, forming distinctive ring complexes (Muñoz et al., 2005). These magmatic rocks, predominantly of Oligocene age, have been interpreted as episodes of submarine and transitional growth in Fuerteventura (Le Bas et al., 1986; Gutiérrez et al., 2006).
In general, outcrops related to the FBC intrusive assemblage exhibit significant variations, and the following four distinct morphologies and characteristic textures can be identified (Fúster et al., 1968; Barrera et al., 1981; Le Bas et al., 1986; Fernández et al., 1997; Mangas et al., 1992, 1994, 1997; Ahijado, 1999; Ancochea et al., 2004; Ahijado et al., 2005; Muñoz et al., 2005):
-
Basaltic, alkaline and carbonatitic dikes and veins of meter scale, decimeter scale, and centimeter scale that are randomly distributed, resulting in a chaotic arrangement (Fig. 2a, b). Related to the carbonatite veins and dikes, an intense fenitization may occur.
-
Shear zones (Fernández et al., 1997), characterized by gradual or diffuse boundaries, which display assimilation structures between different rock bodies, along with the presence of mylonites, and brecciated textures resulting from deformation (Fig. 2c).
-
Pegmatitic textures developed within certain rock bodies, often containing centimeter-sized crystals of rock-forming minerals (Fig. 2d).
-
Contact metamorphism and metasomatism, as well as skarn zones that occur in deformed or undeformed carbonatites, influenced by subsequent hydrothermal fluid circulation (Ahijado et al., 2005; Casillas et al., 2008, 2011).
In addition, during Miocene magmatic pulses, alkaline plutons were formed in the central–western part of Fuerteventura island north of the locality of Pájara (sector 2; Fig. 1). These intrusions constitute typical ring complexes of alkaline magmatic rocks, including nepheline syenites, syenites, and trachytes (Muñoz, 1969). They are regarded as the most recent rocks in the FBC (Fig. 1) and have been dated using the K–Ar method, yielding an approximate age of 20.6 ± 1.7 Ma (Le Bas et al., 1986; Holloway and Bussy, 2008).
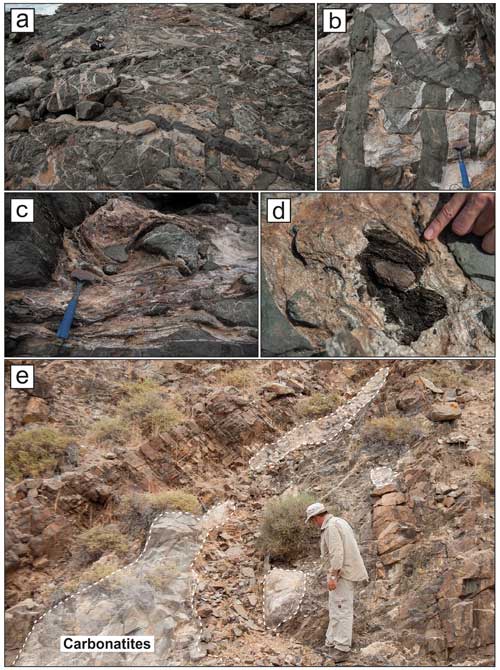
Figure 2(a, b) Images showing typical outcrops of the FBC in the southern area of Ajuy (sector 3; Fig. 1). The images highlight characteristic swarms of alkaline and carbonatitic intrusions (whitish) intersected by later-intruded basaltic dikes (black color). (c) Detailed view of a carbonatitic dike located in a shear zone of sector 3, exhibiting distinct linear sigmoidal structures resulting from deformation. (d) Detailed view of centimeter-sized phlogopite crystals within a carbonatitic dike outcropping in sector 3, displaying a typical pegmatitic texture. (e) Overview of an outcrop of metric-scale carbonatitic dikes in the sector 1 area.
2.2.2 Miocene subaerial volcanic unit
During the Miocene, Fuerteventura witnessed the formation of up to three volcanic edifices (Fig. 1; Coello et al., 1992; Ancochea et al., 1996). The northern volcanic structure, referred to as the Tetir edifice, experienced two volcanic construction phases between 22 and 12.8 Ma (Balcells et al., 1994). These episodes involved the eruption of basalts, picritic basalts, oceanic basalts, trachybasalts, and trachytes. In the central part of the island, the Gran Tarajal edifice developed three different construction phases spanning from 22.5 to 14.5 Ma (Balcells et al., 1994). On the Jandía Peninsula, in the southern part of the island, a volcanic edifice comprising both basaltic and trachybasaltic materials emerged. It formed three successive construction episodes occurring between 20.7 and 14.2 Myr ago (Balcells et al., 1994). Based on their mineralogical and petrological features, the lithologies comprising this unit have not been considered to potentially contain significant concentrations of REEs. Therefore, they have not been included in the present study.
2.2.3 Pliocene and Quaternary volcano sedimentary facies
After the subaerial volcanic activity during the Miocene, a period of volcanic quiescence ensued, leading to the erosion of the previously formed volcanic edifices. Subsequently, during the Pliocene (between 5.3 and 2.6 Ma), a phase of magmatic rejuvenation began, characterized by scattered Strombolian eruptions (Fig. 1). Concurrently, various sedimentary formations emerged across the entire island, including littoral and shallow-water marine deposits, as well as eolian, colluvial, and alluvial subaerial sediments and paleosols from the Pliocene to the Quaternary (Fúster et al., 1968; Zazo et al., 2002; Ancochea et al., 2004).
The soils on Fuerteventura are predominantly classified as eutric Cambisols and lithosols–vitric andosols, according to the FAO/UNESCO (1970) nomenclature. However, the current arid and deforested conditions have led to the extensive erosion of the weathered rock profiles present in different areas of the island. Edaphic calcretes are abundant in Fuerteventura (Chiquet et al., 1999; Alonso-Zarza and Silva, 2002; Huerta et al., 2015; Alonso-Zarza et al., 2020). These formations consist of laminar horizons comprising centimeter-thick layers of micritic calcite, occasionally interspersed with brecciated structures, alongside detrital siliciclastic grains and palygorskite (Alonso and Silva, 2002). Petrological and geochemical analyses suggest that these calcretes originated from eolian dust deposition, with intermittent periods of leaching and calcite precipitation during wetter conditions, wherein biological activity played a significant role in carbonate precipitation (Huerta et al., 2015). Trace element concentrations also indicate that calcium-bearing minerals from the volcanic host rock have a negligible contribution to calcrete formation, with the majority of calcium being supplied by eolian deposits, such as dust from the Sahara and Sahel (Goudie and Middleton, 2001; Menéndez et al., 2007; Scheuvens et al., 2013; Huerta et al., 2015).
3.1 Sampling
Alkaline magmatic rocks and especially carbonatites are considered potential targets for the exploration of REEs (Goodenough et al., 2016; Balaram, 2019; Anenburg et al., 2021; Beland and Jones, 2021). In Fuerteventura, these types of lithologies are found in two distinct geological areas: the Oligocene (sectors 1 and 3; Fig. 1) and the Miocene lithologies related to the FBC (sector 2; Fig. 1).
Considering that weathering profiles may concentrate REE in larger quantities than primary bedrock (Bao and Zhao, 2008; Menéndez et al., 2019; Braga and Biondi, 2023; Chandler et al., 2024), these lithological formations were included in the present study, and sampling was conducted on a selection of six different profiles: (1) Agua Salada ravine (sector 1) and (2) Aulagar ravine (sector 3) hosted by carbonatites, (3) the FV-30 road, (4) Las Peñitas quarry, (5) Palomares ravine, and (6) the Pájara profiles on syenite bedrock (Fig. 1; Table S1 in the Supplement).
Accordingly, a systematic sampling campaign was conducted in three different sectors of Fuerteventura, targeting alkaline and carbonatitic igneous rocks and their associated weathering products. The specific locations of these predetermined sectors are outlined in Fig. 1. As a result, a set of 29 representative samples of potentially REE-enriched magmatic rocks, along with 21 samples of associated weathering products, were collected for further analysis (Table S1). For the weathering products, we conducted six sampling profiles (labeled A to F; Fig. 1) at various suitable points to compare the mineralogical and geochemical changes resulting from weathering of the primary magmatic rocks.
3.2 Petrographic and mineralogical studies
Selected samples of magmatic rocks were prepared in thin sections for textural and mineralogical analysis at the Laboratory of Geological and Paleontological Preparation of the Natural Sciences Museum of Barcelona (LPGiP-MCNB; Barcelona, Spain). A representative subset of these samples was also examined using a JEOL JSM-7100 field emission scanning electron microscope (FE-SEM) at the Scientific and Technological Centers of the Universitat de Barcelona (CCiTUB). The FE-SEM system is equipped with an INCA Pentaflex EDS (energy dispersive spectroscopy) detector (Oxford Instruments, England), which allowed for the acquisition of semi-quantitative analyses of mineral phases. The general operating conditions for the FE-SEM were a 15–20 kV accelerating voltage and a 5 nA beam current.
To achieve accurate and precise mineralogical identification and characterization of the weathering magmatic rocks and calcretes, X-ray powder diffraction (XRPD) measurements were performed using a PANalytical Empyrean powder diffractometer equipped with a PIXcel1D Medipix3 detector at the Integrated X-ray diffraction (XRD) Service of the General Research Support Service of La Laguna University, Spain. The diffractometer employed incident Cu Kα radiation at 45 kV and 40 mA, along with an RTMS (real-time multiple strip) PIXcel1D detector with an amplitude of 3.3473° 2θ. The diffraction patterns were obtained by scanning random powders in the 2θ range from 5 to 80°. Data sets were generated using a scan time of 57 s and a step size of 0.0263° (2θ), with a ° divergence slit. Mineral identification and semi-quantitative results were obtained using PANalytical's HighScore Plus search–match software (v. 4.5) with a PDF+ database.
3.3 Geochemical analyses
The major element composition of carbonates from carbonatites was studied using an electron probe microanalyzer (EPMA) system. The EPMA analyses were conducted on a JEOL JXA-8230 electron microprobe, equipped with five wavelength-dispersive spectrometers and a silicon-drift detector EDS, located at the CCiTUB. The spot mode was employed for the analyses, and the electron column was set to an accelerating voltage of 15 kV and a beam current of 10 nA. Standard counting times of 10 s were used along with a focused beam to achieve the highest possible lateral resolution. The analytical standards employed during the analysis process were celestine (PETJ; Sr Kα), wollastonite (PETL; Ca Kα), periclase (TAPH; Mg Kα), hematite (LiFH; Fe Kα), rhodonite (LiFH; Mn Kα), and albite (TAPH; Na Kα).
Bulk rock geochemical data of major and trace element composition were obtained by X-ray fluorescence (XRF) and inductive coupled plasma (ICP) emission spectrometry. The samples were prepared by lithium metaborate/tetraborate fusion and nitric acid digestion at Actlabs of the Activation Laboratories Ltd. (Ancaster, ON, Canada).
4.1 Petrography and mineralogy
4.1.1 Alkaline magmatic rocks and carbonatites
The primary lithologies under study consist of Oligocene (∼ 25 Ma) alkaline igneous and carbonatitic rocks, as well as Miocene alkaline lithologies (K–Ar age of 20.6 ± 1.7 Ma; Le Bas et al., 1986), that form part of the FBC. Their outcrops extend across kilometer-scale areas but exhibit high heterogeneity at a detailed level due to the occurrence of numerous small intrusions ranging in size from metric to decimetric dimensions (Fig. 2a, b).
At a mineralogical level, separation of the different types of alkaline rocks found in the FBC is complex because these lithologies are intimately associated and infiltrate diffusely, leading to the formation of hybrid intrusions. The materials with the most mafic composition correspond to pyroxenites and melteigites, and their formation is associated with the earliest magmatic fractions. However, these are commonly spatially associated with more differentiated rocks, mainly ijolites, nepheline syenites, and syenites. All these lithologies have a relatively simple mineralogy characterized by varying proportions of nepheline (10 %–30 % modal) and potassium feldspar (50 %–80 % modal) and associated with aegirine-augite and biotite (10 %–30 % modal). A set of accessory minerals with varying proportions (always smaller than 5 % modal) also occurs, including ilmenite, titanite, zircon, and fluorapatite.
At a textural level, the alkaline series lithologies of the FBC present granular textures with millimeter-sized euhedral grains. However, in some of the intrusions in sectors 2 and 3, pegmatitic syenites-ijolites were detected with centimeter-sized grains characterized by the presence of large aegirine-augite crystals.
Some of the intrusions described in the three sectors show aphanitic textures caused by faster cooling, resulting in rocks with similar mineralogy but extrusive-type textural characteristics. Therefore, due to their textural features, some dikes and apophyses, although mineralogically equivalent, should be classified as trachytes and phonolites.
Carbonatitic intrusions commonly co-occur with the alkaline rocks, sharing similar morphology, textures, and spatial distribution within the outcrops (Fig. 2e). Furthermore, alkaline and/or carbonatitic intrusions can be occasionally associated with mafic intrusions, primarily pyroxenites, and alkaline gabbros. In addition, a subsequent set of mafic dikes with basaltic composition overlaps the previous intrusive bodies (Fig. 2a, b).
All carbonatites described in different outcrops from sectors 1 and 3 are predominantly composed of calcite (95 % modal) and can thus be classified as calciocarbonatites (Le Maitre et al., 2005). None of the studied samples shows the occurrence of ferromagnesian carbonates such as ankerite, dolomite, and/or siderite, as well as REE carbonates. Texturally, calcite occurs as euhedral crystals ranging in size from millimeters to centimeters, often recrystallized and exhibiting polysynthetic twinning. In some cases, a secondary micritic calcite matrix is present, filling interstitial spaces and fractures.
The major element composition of calcite is relatively consistent across all the carbonatite samples. Notably, there are significant contents of SrO, with values of up to 5.43 wt %, while REEs are absent from the carbonate composition (Table S2).
The accessory mineralogy (∼ 5 % modal) comprises disseminated phases within the calcium carbonate. Among them, the occurrence of minerals from the spinel group, including magnetite (Fig. 3a), and primarily jacobsite, occurring as subhedral crystals of up to 50 µm (Fig. 3b). Another characteristic mineral is perovskite, occurring as subhedral crystals of up to 100 µm. These grains are remarkable for their significant Nb contents, as described in other carbonatitic localities worldwide (Torró et al., 2012). Britholite also occurs as subhedral crystals of up to 100 µm (Fig. 3b). This primary britholite contains significant LREE content (Fig. 4), and its alteration leads to the formation of secondary REE-enriched phosphates, mainly monazite–(Nd) (Fig. 3c), which also contains substantial amounts of La and Ce (Fig. 4). REEs, in addition to occurring in primary britholite and secondary monazite, were also detected in tiny pyrochlore grains heterogeneously disseminated in the calcite groundmass (Fig. 3b). In some cases, pyrochlore forms euhedral crystals of up to 20 µm, also included in calcite (Fig. 3d). This pyrochlore shows slight zoning towards plumbopyrochlore (Christy and Atencio, 2013), with significant enrichment in Pb observed at grain borders (Fig. 3d).
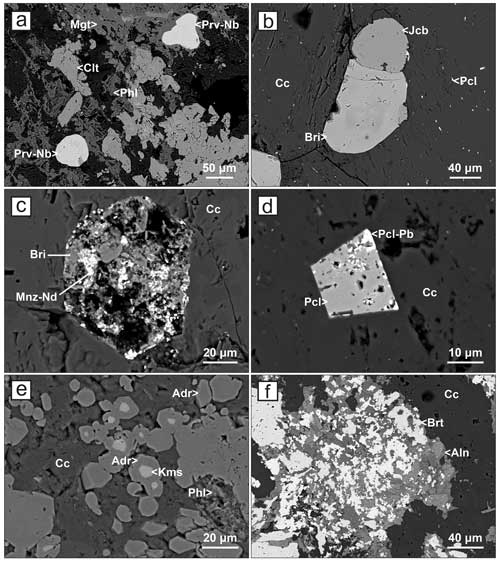
Figure 3SEM (backscattered electron, BSE) images of the Fuerteventura carbonatites. (a) Subhedral crystals of niobium-rich perovskite (Prv–Nb) associated with phlogopite (Phl) and magnetite (Mgt) aggregates. The association has been affected by secondary hydrothermal processes, leading to the formation of celestine (Clt). (b) Typical subhedral crystal of jacobsite (Jcb) associated with britholite (Bri). Both crystals are hosted in magmatic calcite (Cc), with numerous disseminated microcrystals of pyrochlore (Pcl). (c) Partially altered subhedral grain of britholite (Bri) hosted in magmatic calcite (Cc). The alteration process led to the formation of secondary REE phosphates such as monazite–(Nd) (Mnz–Nd). (d) Euhedral crystal of pyrochlore (Pcl) hosted in calcite (Cc). Brighter areas developed on the grain's borders correspond to plumbopyrochlore (Pcl–Pb) zonation. (e) Typical mineral association related to small skarn-like areas associated with carbonatites. Subhedral zoned crystals of andradite (Adr), hosted in calcite (Cc) and phlogopite (Phl), with a significant Zr zoning leading to kerimasite (Kms) cores. (f) Typical low-metamorphic alteration developed on carbonatites composed of allanite (Aln) aggregates hosted in calcite (Cc) and associated with secondary baryte (Brt). Abbreviations of mineral names in all the pictures follow the criteria proposed by Warr (2021).
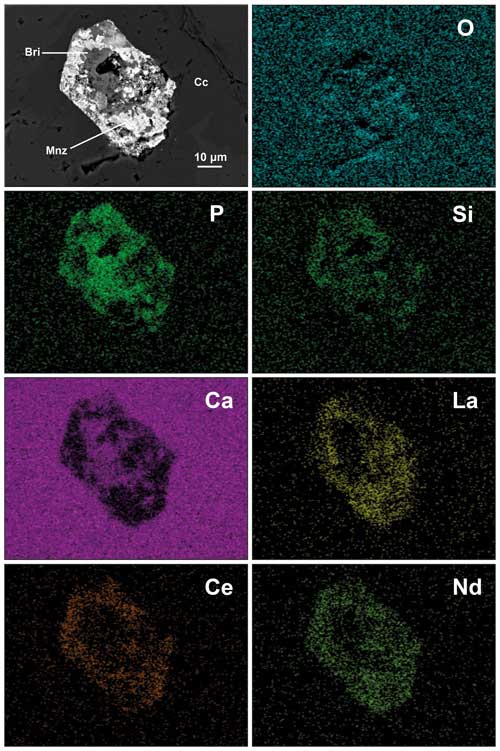
Figure 4Wavelength-dispersive X-ray maps of representative compositional elements for an altered grain of britholite (Bri) hosted in calcite (Cc) and partially transformed into secondary monazite (Mnz).
Carbonatites can be affected by certain contact metamorphism, especially in sectors 1 and 3 (Fig. 1), and may exhibit a slightly different mineralogy from the one described thus far. This is characterized by the occurrence of skarn-type metamorphic minerals formed due to the interaction between carbonatites and spatially associated silica-rich rocks. Among these minerals, there are subhedral crystals of andradite, up to 30 µm in size, implanted in a matrix of secondary calcite and phlogopite, exhibiting pronounced zoning with kerimasite cores (Fig. 3e). In these areas, the occurrence of REE mineralizations associated with allanite (Fig. 3f) is also typical. Allanite occurs as granular aggregates associated with hydrothermal secondary sulfates, primarily baryte (Fig. 3f) but occasionally celestine (Fig. 3c).
This particular mineralogy, typically associated with skarn formations, emerges from the interaction between a carbonatite intrusion and surrounding silicate rocks, in contrast to the typical process. It has recently gained attention from several researchers in various carbonatite locations worldwide, who have coined the term anti-skarn to describe it (Anenburg and Mavrogenes, 2018; Yaxley et al., 2022).
4.1.2 Weathering products
In certain areas within the three studied sectors (Fig. 1), there is evidence of the development of characteristic shallow geological formations consistently associated with weathering, which affect the outlined magmatic lithologies (Figs. 5, 6). These geological products were studied through the analysis of six alteration profiles developed on carbonatites (Agua Salada and Aulagar) and syenites (Palomares ravine, FV-30 road, Las Peñitas quarry, and Pájara) (Fig. 1).
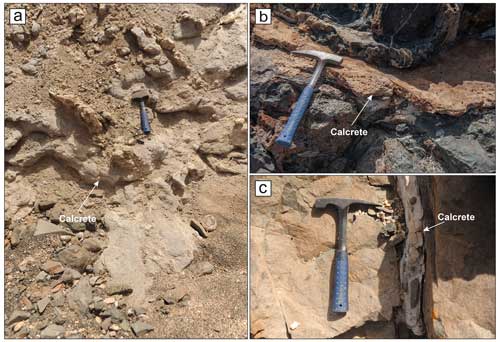
Figure 5(a) General view of a typical surface outcrop of Quaternary calcrete located in the Aulagar ravine area (profile B, sector 3; Fig. 1). (b) Centimeter-thick calcrete layer filling a fracture between two carbonatitic dikes in the Aulagar ravine area (profile B, sector 3; Fig. 1). (c) Calcrete layer developed within fractures between carbonatitic rocks in the Agua Salada ravine area (profile A, sector 1; Fig. 1).
The calcrete and carbonatite section generally consist of centimeter-scale calcrete veins injected into the carbonatitic host rock (Fig. 5). In general, the development of weathering products was not detected on carbonatites in any of the studied sectors of the FBC.
Weathering products developed on syenite bedrock are generally more abundant. The cambic B horizon displays reddish to yellowish colorations (5YR6/6), with a thickness of up to 20–30 cm. Additionally, it is common to find BC horizons instead of B horizons, while the C horizon is well-developed, reaching a 30–40 cm thickness at certain levels of the profile (Fig. 6). Furthermore, except for the Las Peñitas profile (E profile; Fig. 1), centimeter-scale calcrete bands (Bk; Jahn et al., 2006) were also detected in deeper layers across all the studied profiles.
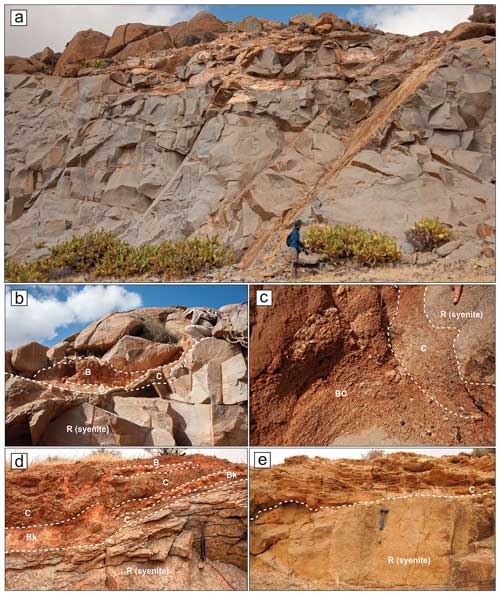
Figure 6(a) General view of Las Peñitas quarry syenite outcrop (profile E, sector 2; Fig. 1), where it is possible to distinguish different fractures filled by injected secondary weathering products. (b) Syenite weathering profile in Las Peñitas quarry (profile E, sector 2; Fig. 1) showing surface erosion and B, BC, and C horizons injected in the syenite bedrock (R). (c) Weathering profile displaying the development of C and BC horizons associated with a syenite protolith (R), located in Las Peñitas quarry (profile E, sector 2; Fig. 1). (d) Weathering profile developed on syenite in the FV-30 road area (profile D, sector 2) exhibiting the development of C, B, and calcrete (Bk) horizons. (e) Weathering profile on syenite protolith (R) displaying a metric-sized C horizon in the Pájara area (profile F, sector 2; Fig. 1).
In terms of mineralogical composition, carbonatite profiles exhibit significant changes due to weathering. In general, weathering processes lead to a reduction in calcite, the disappearance of fluorapatite, and the formation of secondary minerals like palygorskite (Fig. 7). The contribution from lateral slope movement is also evident through the presence of residual plagioclase and clinopyroxene.
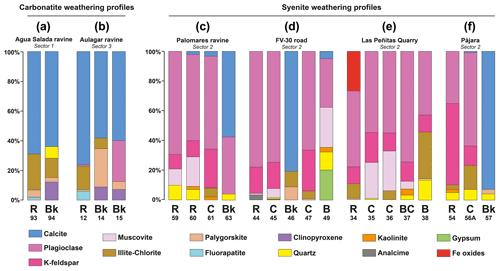
Figure 7Graphical mineralogical quantification of the studied weathering profiles: (a) Agua Salada ravine, (b) Aulagar ravine, (c) Palomares ravine, (d) FV-30 road, (e) Las Peñitas quarry, and (f) Pájara. The corresponding class assigned to the edaphic horizons (B, BC, Bk, C, and R) and the sample number are shown at the foot of the columns.
In the case of syenite weathering profiles, illite/chlorite and kaolinite are the predominant secondary products, followed by muscovite and palygorskite (Fig. 7). Other minerals such as quartz were also detected, even in the C horizons.
4.2 Bulk rock and mineral geochemistry
Chemical analysis of the major, minor, and trace elements were carried out in order to evaluate the geochemical features and the distribution of REEs on 25 representative samples of igneous rocks from the FBC, including trachytes, phonolites, syenites, ijolites, and carbonatites (Table S3). In addition, we also analyzed 21 samples of weathering products (Table S4).
The total REY (REEs plus yttrium) content in the FBC igneous rocks exhibits widespread and significant enrichment in comparison to the average crustal values (∼ 125 ppm; Rudnick and Gao, 2014). Notably, the extrusive and magmatic alkaline lithologies (trachytes and phonolites, as well as syenites and ijolites) show variable REY values ranging between about 230 and 1400 ppm (Table S3). In contrast, the carbonatitic rocks exhibit REY content more than 10 times greater than the alkaline lithologies, with specific samples reaching maximum values of up to about 10 300 ppm, as evidenced in sample 85a sourced from a carbonatite outcrop in sector 1 (Table S3).
The weathered magmatic rocks, though moderately significant in REY content relative to the average crustal values (Table S4), still exhibit slightly lower levels compared to the content observed in the associated alkaline and carbonatitic protoliths (Table S3). A contrasting pattern emerges in the calcretes, where REY values experience a sharp reduction, presenting virtually negligible values ranging between 20 and 72 ppm REY. These levels are significantly below the average Earth's crust values (Rudnick and Gao, 2014) and are markedly lower than those observed in both the alkaline lithologies and, particularly, the carbonatites of the FBC.
REE-normalized diagrams further underscore this distribution, portraying elevated content in the carbonatites, followed by the alkaline rocks (Fig. 8a). Meanwhile, the weathered magmatic rocks and calcretes (Fig. 8b) display significantly lower values. All studied lithologies exhibit clear negative patterns, indicative of enrichment in LREEs relative to HREEs. Notably, carbonatites and alkaline rocks (Fig. 8a) exhibit a flattening of these negative patterns in the final segment, indicating a certain degree of HREE enrichment.
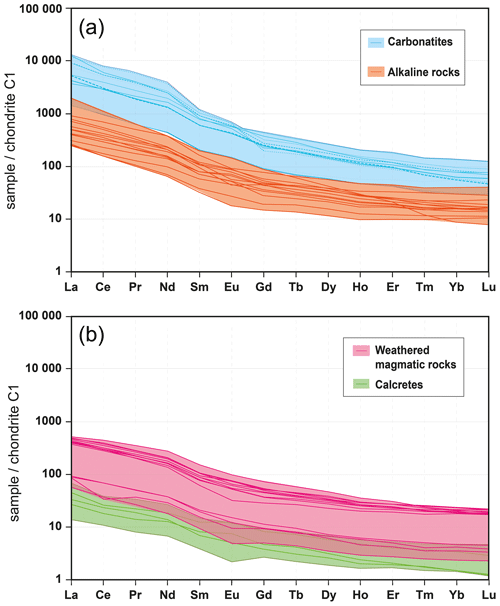
Figure 8REE plots of the studied Fuerteventura lithologies normalized to C1 chondrites. Normalization values are from McDonough and Sun (1995).
The FBC carbonatites exhibit a depletion in some critical elements commonly associated with this lithology such as Nb or Ta (Table S3). Negative anomalies of both Nb and Ta are clearly observed in the multi-element diagrams of carbonatite samples (Fig. 9a). However, given the presence of pyrochlore in the carbonatites, these anomalies in Nb and Ta are likely not indicative. We interpret that the low concentrations of these elements could be attributed to an analytical artifact that would underestimate the contents of high field strength elements (HFSEs) due to the challenge of pyrochlore dissolution in the analytical digestion protocols employed. These protocols have been primarily devised to assess the contents of REEs rather than HFSEs. Additionally, alkaline rock patterns also show a distinctive negative anomaly in Sr (Fig. 9b). As for the weathering products, their contents of other minor elements do not indicate significant concentrations of metals or critical elements like Nb or Ta (Table S4). The multi-element diagrams for the calcretes exhibit a negative Ta anomaly (Fig. 9c), while the patterns of weathered magmatic rocks do not reveal notable anomalies in any group of elements (Fig. 9d).
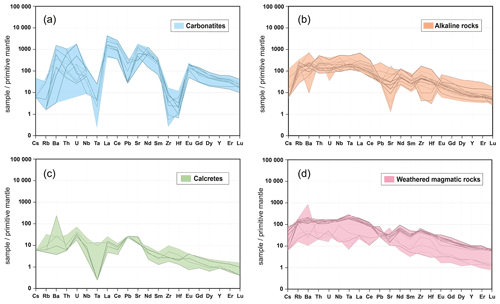
Figure 9Multi-elemental trace element plots of Fuerteventura intrusive lithologies normalized to the primitive mantle. Normalization values from McDonough and Sun (1995).
A specific geochemical study of REE distribution in the six studied weathering profiles was also conducted (Fig. 10). The main objective was to evaluate the geochemical interactions between the protolith and the related weathering lithologies, with the aim of detecting potential REE enrichments or depletions caused by weathering processes.
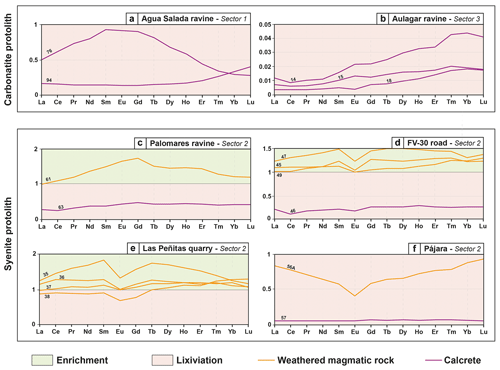
Figure 10REE weathering enrichment/leaching diagrams between primary magmatic protoliths (carbonatites and syenites) and the associated weathering products from the studied profiles (Fig. 1). The sample number is labeled on the corresponding pattern line.
In the exchange patterns of calcretes occurred within carbonatitic host rocks, as analyzed in the Agua Salada and Aulagar ravine profiles (Figs. 10a, b), REE concentrations are 2 orders of magnitude lower than in the carbonatite (Fig. 10a), as also previously determined from the REE diagrams (Fig. 8). Notably, it was observed that the concentration of REEs is directly correlated with the distance from the host rock. This is evident in calcrete sample 14 (Fig. 10b), which is situated closer to the carbonatites and exhibits a slight enrichment in REE concentrations (∼ 51.3 ppm ΣREY; Table S4) compared to samples 15 and 18 (Fig. 10b), which are located farther away from the carbonatite host rock and show a slight depletion in REE (∼ 20.1 to ∼ 32.6 ppm ΣREY; Table S4). In addition, although the values of all elements are depleted in the calcrete patterns, there is a greater depression in LREE than in HREE relative to the protolith, resulting in typically positive patterns, except for sample 76 from the Agua Salada ravine, where a clear inverse trend is observed (Fig. 10a).
In general, the diagrams in Fig. 10 show that weathering products on syenites exhibit enrichment relative to the protolith (green areas in Fig. 10c, d, e). However, calcrete samples, whether developed on carbonatites or syenites, consistently show depletions compared to the protolith contents (reddish areas in Fig. 10c, d, f). The diagrams corresponding to the weathering products generated on syenites exhibit similar morphologies (Fig. 10c, d, e, f). Overall, these lithologies are characterized by enrichment in REEs relative to the protolith, as well as V-shaped patterns, featured by the presence of a negative anomaly in Eu, which is also reported in all C and B horizons developed on syenites, except sample 61 (Fig. 10c), and is likely related to plagioclase crystallization.
5.1 REE distribution on the FBC magmatic rocks
The FBC magmatic rocks in the three study sectors encompass alkaline lithologies (trachytes, phonolites, syenites, melteigites, and ijolites), as well as carbonatites. Regarding the group of alkaline rocks, the detected REE content varies between 214 and 1330 ppm (Table S3), significantly higher than the average concentration determined in the Earth's crust (∼ 125 ppm; Rudnick and Gao, 2014). However, this finding is not surprising, and the observed values in Fuerteventura are not anomalous as these types of lithologies typically exhibit REE concentrations within this range (Dostal, 2017). Therefore, the measured REE concentrations are neither significant nor sufficiently elevated to hypothetically consider these lithologies as a potential non-conventional deposit of these critical elements in the FBC.
On the other hand, FBC carbonatites present significantly higher values in terms of REE content. In the studied carbonatite samples from sectors 1 and 3 (carbonatites do not outcrop in sector 2), REE content ranges between about 1300 and 10 300 ppm. The latter value corresponds to the richest REE-detected sample in the entire FBC, which is located in the Agua Salada ravine area of sector 1 (Table S3; Fig. 1).
The reported REE content values in the FBC carbonatites are similar to the general average concentrations found in other locations worldwide where carbonatites are exploited for REE extraction. This is the case, for example, for Bayan Obo, the largest REE deposit in the world (Lai et al., 2015; Liu et al., 2018). In this locality, high-grade carbonatites exhibit average concentrations of 2880 ppm (Wu, 2008; Smith et al., 2015, 2016), which are equivalent to those measured in some of the samples from Fuerteventura. It should be noted that low-grade carbonatite ore from Bayan Obo presents extremely high values in comparison to the FBC, with REE concentrations reaching 30 750 ppm (Chao et al., 1997; Smith et al., 2016).
Another significant example is the Mountain Pass carbonatite in California, USA (Olson et al., 1954; Haxel, 2005). In this REE deposit, the average values across the whole complex are around 2580 ppm (Castor, 2008; Mariano and Mariano, 2012; Smith et al., 2016), also in line with REE concentrations detected in the present study for the FBC carbonatites.
This comparative analysis can also be carried out using normalized REE values (Fig. 11). In this regard, FBC carbonatites are significantly depleted in LREE compared to those from Bayan Obo (Yang et al., 2019) and Mountain Pass (Castor et al., 2008), although they show similar values to other REE deposits associated with carbonatites, such as those in Ashram, Canada (Beland and Jones, 2021), and Bear Lodge, USA (Moore et al., 2015; Smith et al., 2016; Fig. 11). However, the pattern of the Fuerteventura carbonatites exhibits a slightly less pronounced slope, indicating a higher relative content of HREEs, which are considered the materials with the highest risk of supply among all the critical raw materials (CRMs) defined by the EC (European Commission, 2023a). In fact, in the FBC carbonatites, the normalized HREE values are equivalent to those reported in the primary carbonatitic rocks from the deposits of Bayan Obo (China) and Mountain Pass (USA) (Fig. 11). The relative significant HREE content reported in FBC carbonatites holds particular significance. The use of HREEs, such as Yb, Er, and Tm, is of particular interest in cutting-edge photonic and nanotechnology applications.
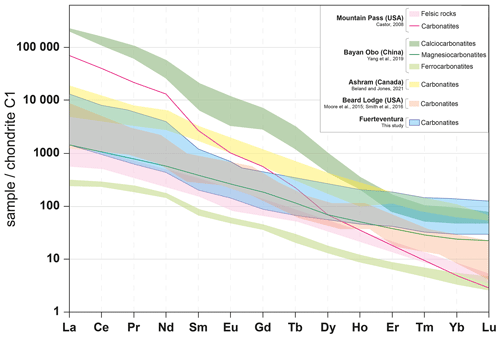
Figure 11REE plot of the studied Fuerteventura carbonatites compared to other carbonatitic localities worldwide where REE deposits have been reported. REE contents for comparison are from Castor (2008), Yang et al. (2019), and Beland and Jones (2021). Normalization values are from McDonough and Sun (1995).
At the mineralogical level, it was observed that, in the FBC carbonatites, the main REE-hosting minerals are accessory phases and are primarily minerals from the pyrochlore group found as disseminated euhedral micro-crystals implanted in primary calcite (Fig. 3b, d). Another REE-bearing mineral in the FBC carbonatites is britholite, which exhibits significant LREE content. However, this mineral is commonly altered to monazite (Figs. 3c, 4), interpreted as a secondary phase but also a carrier of these critical elements (Chen et al., 2017).
Another noteworthy aspect is the lack of REE fluorcarbonates like bastnäsite REE(CO3)F, parisite Ca(REE)2(CO3)3F2, synchysite Ca(REE)(CO3)2F, or huanghoite Ba(REE)(CO3)2F. They do not occur in the FBC as they do in other REE deposits associated with, for example, the Bayan Obo carbonatite or the Sulphide Queen carbonatite from Mountain Pass (Castor et al., 2008; Smith et al., 2015, 2016).
5.2 REE distribution on the associated weathering products
The weathering materials developed on magmatic rocks, also analyzed for their REE concentrations, constitute the remnants of soils that were interpreted as developed under wetter conditions during a humid phase of the oxygen isotope stage 2, spanning from 29 000 to 20 000 years BP (Huerta et al., 2015). This period aligns with the Last Glacial Maximum, marked by heightened humidity in the Canary Islands, resulting in slope erosion and the formation of talus flatiron (Gutiérrez-Elorza et al., 2013). Over time, these materials have undergone substantial volume reduction due to human-driven deforestation and erosion, primarily before the 15th century (Machado-Yanes, 1996). Notably, topography plays an essential role in the distribution of these weathering profiles and influences specific physical attributes such as slope (FAO/UNESCO, 1974).
The studied weathering products developed on syenite rocks (profiles C, D, E, and F; Figs. 1, 7) are classified by FAO/UNESCO (1974) as eutric Cambisols, reflecting a Mediterranean climate condition. Indeed, on the African continent, which is adjacent to the Canary Islands, eutric Cambisols are primarily found within the tropical subhumid zone, gradually transitioning into the semi-arid zone (FAO/UNESCO, 1974). These syenite weathering profiles exhibit better-preserved characteristics and a more significant extent compared to those studied in carbonatites (profiles A and B; Figs. 1, 7). In general, intensive weathering plays a crucial role in the formation of REE deposits, as these elements tend to be concentrated in such geological formations compared to others leached during the weathering process. This phenomenon is exemplified in several locations worldwide where REE deposits associated with weathering products occur; for instance, Bear Lodge in the USA (Andersen et al., 2017), Chuktukon and Tomtor in Russia (Kravchenko and Pokrovsky, 1995; Kravchenko et al., 2003; Chebotarev et al., 2017), Las Mercedes in the Dominican Republic (Torró et al., 2017), Araxá in Brazil (Braga and Biondi, 2023), and Mount Weld in Australia (Zhukova et al., 2021; Chandler et al., 2024), among many others. However, the weathering processes on Fuerteventura are characterized by fluctuating climatic conditions and intense erosion in the context of a typical Mediterranean climate, which is in turn characterized by drier conditions and a lower propensity for intense weathering compared to tropical climates. The weathering processes on Fuerteventura do not therefore typically lead to the development of laterites and mature weathering profiles, since these conditions do not favor the formation and subsequent preservation of these products.
5.3 Fuerteventura carbonatites as potential REE source
Based on the mineralogical and geochemical data, it can be concluded that, among the lithologies studied in the FBC, only the carbonatites are favorable targets for REEs exploration. Therefore, the primary alkaline rocks, as well as the entire suite of corresponding secondary weathering products, can be ruled out.
The geochemical data obtained from the oceanic carbonatites of Fuerteventura, exemplified in multielement and REE diagrams (Fig. 8), suggest a petrogenetic affinity with carbonatites associated with intracontinental rift geological settings. This similarity has also been previously highlighted by other authors such as Carnevale et al. (2021), who, based on stable isotope data (δ13C and δ18O) and noble gases isotopic composition (He, Ne, and Ar), suggested that oceanic and continental carbonatites were comparable in petrogenetic terms. Therefore, despite the lingering questions about the formation processes of oceanic carbonatites, their assessment as a possible source of critical metals, especially REEs, could be considered in the same way as their continental counterparts.
However, when considering a more detailed assessment of the sectors where the FBC carbonatites outcrop, it is essential to note that the distribution of these outcrops and thus potential REE mineralization is not straightforward. The carbonatite outcrops have a very limited surface distribution on the order of meters (Fig. 2e) and exhibit complex structural features influenced by shear metamorphism (Fig. 2c) and overlapping episodes of intrusive activity that resulted in swarms of dikes with intricate distributions (Fig. 2a, b). Hence, these general features of the carbonatite outcrops make it imperative to validly estimate their volume and to carry out more precise studies of their depth distribution.
Then, it is important to highlight that any attempt to assess potential REE deposits linked to FBC carbonatites must consider the irregular distribution of these mineralizations. In addition, it should also be considered the existence of regulatory constraints that may stem from the allocation of land for strategic military activities, as well as environmental considerations to safeguard natural and marine–coastal areas, especially bearing in mind that Fuerteventura is a UNESCO biosphere reserve territory. This latter point is particularly pertinent for a specific area within sector 3 (Fig. 1). Therefore, any comprehensive analysis of the potential of FBC carbonatites as REE sources must also factor in these potential restrictions tied to land use regulations aimed at upholding the broader socioeconomic, environmental, and societal interests inherent to a distinctive site like the island of Fuerteventura.
A preliminary study of the distribution of REEs was conducted through mineralogical and geochemical analyses of alkaline and carbonatitic igneous rocks within the FBC, along with associated weathering products. Based on the gathered data and their corresponding interpretations, our findings can be summarized as follows:
- i.
The concentrations of REEs present in the alkaline and carbonatitic rocks of the FBC are significant and exceed the average values attributed to the Earth's crust.
- ii.
The weathering products developed on these magmatic rocks do not exhibit significant REE enrichment.
- iii.
Calcified horizons (Bk and calcretes) have practically negligible concentrations of REE elements that are consistent with their sedimentary and eolian origin reported in the previously published literature. Colluvial processes may have influenced the lateral transport and accumulation of REEs in Pleistocene–Holocene deposits distant from the source area.
- iv.
The detected concentrations of REY in carbonatites range up to about 10 300 ppm, which is a comparable concentration to other locations hosting significant deposits of these critical elements worldwide.
- v.
Within carbonatites, REEs are primarily hosted in two accessory mineral phases: (1) oxides belonging to the pyrochlore group and (2) phosphates. In this second group, primary phases such as REE-bearing britholite can be distinguished, as well as monazite generated as a secondary product from the britholite alteration.
- vi.
Primary calcite in the Fuerteventura carbonatites is not the predominant host of REEs. It displays a highly homogeneous composition with insignificant Fe–Mg content and negligible REEs.
- vii.
The complex structural features of the studied FBC outcrops (deformation, metamorphism, swarms of dikes from different intrusive pulses, etc.) make it essential to conduct more detailed studies to quantify real REE resources.
- viii.
All the studied sectors contain outcrops located in restricted areas due to environmental or military use concerns. Any further detailed analysis of REE distribution in the FBC carbonatites must take into account the environmental, socioeconomic, and geostrategic factors.
The authors confirm that the data supporting the findings of this study are available within this article and Table S1.
The supplement related to this article is available online at: https://doi.org/10.5194/se-15-639-2024-supplement.
Conceptualization: MC, IM, LQ, JY, and JM. Fieldwork and sampling: MC, IM, LQ, JY, RC, AA, and JM. Methodology: MC, IM, JY, and JM. Validation of results: MC, IM, LQ, JY, RC, JMR, and JM. Data curation: MC, IM, and JM. Writing (original draft preparation): MC, IM, JY, and JM. Writing (review and editing): MC, IM, LQ, JY, RC, JMR, and JM. Supervision: IM, JY, and JM. Project administration: JMR and JM. Funding acquisition: IM, JY, RC, JMR, and JM.
The contact author has declared that none of the authors has any competing interests.
Publisher's note: Copernicus Publications remains neutral with regard to jurisdictional claims made in the text, published maps, institutional affiliations, or any other geographical representation in this paper. While Copernicus Publications makes every effort to include appropriate place names, the final responsibility lies with the authors.
The collection of samples in specific protected areas required authorization from the Fuerteventura Government. We appreciate the cooperation and assistance provided by the Spanish Army, especially by the soldier Liberto Yeray Puga Acosta, who facilitated our access to the Pájara CMT restricted military area to carry out sampling. We thank Gerard Lucena from the LPGiP-MCNB for his thorough work in the elaboration of polished thin sections. We would also like to express our acknowledgements to Michael Anenburg, an anonymous reviewer, and editors Johan Lissenberg and Andrea Di Muro for their constructive and enriching comments and corrections which greatly improved the initial version of this work.
This research has been supported by the Agencia Canaria de Investigación, Innovación y Sociedad de la Información (grant nos. ENE2013SD-22/25 (Tierras Raras project) and ProID-20211010027 (MAGEC-REEmounts project)) and the Ministerio de Ciencia e Innovación (grant nos. ENE2013-47826-C4-4-R, ENE2016-74889-C4-2-R, and CGL2016-75062-P).
This paper was edited by Johan Lissenberg and reviewed by Michael Anenburg and one anonymous referee.
Acosta-Mora, P., Domen, K., Hisatomi, T., Lyu, H., Méndez-Ramos, J., Ruiz-Morales, J. C., and Khaidukov, N. M.: “A bridge over troubled gaps”: up-conversion driven photocatalysis for hydrogen generation and pollutant degradation by near-infrared excitation, Chem. Commun., 54, 1905–1908 https://doi.org/10.1039/C7CC09774C, 2018.
Aiglsperger, T., Proenza, J. A., Lewis, J. F., Labrador, M., Svojtka, M., Rojas-Purón, A., Longo, F., and Ďurišová, J.: Critical metals (REE, Sc, PGE) in Ni laterites from Cuba and the Dominican Republic, Ore Geol. Rev., 73, 127–147, https://doi.org/10.1016/j.oregeorev.2015.10.010, 2016.
Ahijado, A.: Las intrusiones plutónicas e hipoabisales del sector meridional del Complejo Basal de Fuerteventura, Doctoral Thesis, Universidad Complutense de Madrid, 392 pp., 1999.
Ahijado, A., Casillas, R., Nagy, G., and Fernández, C.: Sr-rich minerals in a carbonatite skarn, Fuerteventura, Canary Islands (Spain), Mineral. Petrol., 84, 107–127, https://doi.org/10.1007/s00710-005-0074-8, 2005.
Alonso, E., Sherman, A. M., Wallington, T. J., Everson, M. P., Field, F. R., Roth, R., and Kirchain, R. E.: Evaluating Rare Earth Element Availability: A Case with Revolutionary Demand from Clean Technologies, Environ. Sci. Technol., 46, 3406–3414, https://doi.org/10.1021/es203518d, 2012.
Alonso-Zarza, A. M. and Silva, P. G.: Quaternary laminar calcretes with bee nests evidences of small-scale climatic fluctuations, Eastern Canary Islands, Spain, Palaeogeogr. Palaeocl., 178, 119–135, https://doi.org/10.1016/S0031-0182(01)00405-9, 2002.
Alonso-Zarza, A. M., Rodríguez-Berriguete, Á., Casado, A. I., Martín-Pérez, A., Martín-García, R., Menéndez, I., and Mangas, J.: Unravelling calcrete environmental controls in volcanic islands, Gran Canaria Island, Spain, Palaeogeogr. Palaeocl., 554, 109797, https://doi.org/10.1016/j.palaeo.2020.109797, 2020.
Ancochea, E., Brändle, J. L., Cubas, C. R., Hernán, F., and Huertas, M. J.: Volcanic complexes in the eastern ridge of the Canary Islands: the Miocene activity of the Island of Fuerteventura, J. Volcanol. Geoth. Res., 70, 183–204, https://doi.org/10.1016/0377-0273(95)00051-8, 1996.
Ancochea, E., Barrera, J. L., and Bellido, F.: Canarias y el vulcanismo neógeno peninsular. Geología de España, 635–682, in: Fuentes mantélicas y evolución del volcanismo canario, edited by: Aparicio, A., Hernán, F., Cubas, C. R., and Araña, V., 2003, Estud. Geol.-Madrid, 59, 5–13, https://doi.org/10.3989/egeol.03591-477, 2004.
Andersen, A. K., Clark, J. G., Larson, P. B., and Donovan, J. J.: REE fractionation, mineral speciation, and supergene enrichment of the Bear Lodge carbonatites, Wyoming, USA, Ore Geol. Rev., 89, 780–807, https://doi.org/10.1016/j.oregeorev.2017.06.025, 2017.
Anenburg, M. and Mavrogenes, J. A.: Carbonatitic versus hydrothermal origin for fluorapatite REE-Th deposits: experimental study of REE transport and crustal “antiskarn” metasomatism, Am. J. Sci., 318, 335–366, https://doi.org/10.2475/03.2018.03, 2018.
Anenburg, M., Broom-Fendley, S., and Chen, W.: Formation of Rare Earth Deposits in Carbonatites, Elements, 17, 327–332, https://doi.org/10.2138/gselements.17.5.327, 2021.
Balcells, R., Barrera, J. L., Gómez, J. A., Cueto, L. A., Ancochea, E., Huertas, M. J., Ibarrola, E., and Snelling, N.: Edades radiométricas en la Serie Miocena de Fuerteventura (Islas Canarias), Bol. Geol. Min., 35, 450–470, 1994.
Balaram, V.: Rare earth elements: A review of applications, occurrence, exploration, analysis, recycling, and environmental impact, Geosci. Front., 10, 1285–1303, https://doi.org/10.1016/j.gsf.2018.12.005, 2019.
Balogh, K., Ahijado, A., Casillas, R., and Fernández, C.: Contributions to the chronology of the Basal Complex of Fuerteventura, Canary Islands, J. Volcanol. Geoth. Res., 90, 81–101, https://doi.org/10.1016/S0377-0273(99)00008-6, 1999.
Bao, Z. and Zhao, Z.: Geochemistry of mineralization with exchangeable REY in the weathering crusts of granitic rocks in South China, Ore Geol. Rev., 33, 519–535, https://doi.org/10.1016/j.oregeorev.2007.03.005, 2008.
Barrera, J. L., Fernândez-Santín, S., Fúster, J. M., and Ibarrola, E.: Ijolitas-Sienitas-Carbonatitas de los Macizos del Norte de Fuerteventura, Bol. Geol. Min., 92, 309–321, 1981.
Barteková, E. and Kemp, R.: National strategies for securing a stable supply of rare earths in different world regions, Resour. Policy, 49, 153–164, https://doi.org/10.1016/j.resourpol.2016.05.003, 2016.
Beland, C. M. J. and William-Jones, A. E.: The mineralogical distribution of the REE in carbonatites: A quantitative evaluation, Chem. Geol., 585, 120558, https://doi.org/10.1016/j.chemgeo.2021.120558, 2021.
Berger, A., Janots, E., Gnos, E., Frei, R., and Bernier, F.: Rare earth element mineralogy and geochemistry in a laterite profile from Madagascar, Appl. Geochem., 41, 218–228, https://doi.org/10.1016/j.apgeochem.2013.12.013, 2014.
Borst, A. M., Smith, M. P., Finch, A. A., Estrade, G., Villanova-de-Benavent, C., Nason, P., Marquis, E., Horsburgh, N. J., Goodenough, K. M., Xu, C., Kynický, J., and Geraki, K.: Adsorption of rare earth elements in regolith-hosted clay deposits, Nat. Commun., 11, 4386, https://doi.org/10.1038/s41467-020-17801-5, 2020.
Braga, J. M. and Biondi, J. C.: Geology, geochemistry, and mineralogy of saprolite and regolith ores with Nb, P, Ba, REEs (+ Fe) in mineral deposits from the Araxá alkali-carbonatitic complex, Minas Gerais state, Brazil, J. S. Am. Earth Sci., 125, 104311, https://doi.org/10.1016/j.jsames.2023.104311, 2023.
Braun, J. J., Pagel, M., Herbilln, A., and Rosin, C.: Mobilization and redistribution of REEs and thorium in a syenitic lateritic profile: A mass balance study, Geochem. Cosmochim. Ac., 57, 4419–4434. https://doi.org/10.1016/0016-7037(93)90492-F, 1993.
Carnevale, G., Caracausi, A., Correale, A., Italiano, L., and Rotolo, S. G.: An Overview of the Geochemical Characteristics of Oceanic Carbonatites: New Insights from Fuerteventura Carbonatites (Canary Islands), Minerals, 11, 203, https://doi.org/10.3390/min11020203, 2021.
Casillas, R., Nagy, G., Demény, A., Ahijado, A., and Fernández, C.: Cuspidine–niocalite–baghdadite solid solutions in the metacarbonatites of the Basal Complex of Fuerteventura (Canary Islands), Lithos, 105, 25–41, https://doi.org/10.1016/j.lithos.2008.02.003, 2008.
Casillas, R., Démeny, A., Nagy, G., Ahijado, A., and Fernández, C.: Metacarbonatites in the Basal Complex of Fuerteventura (Canary Islands). The role of fluid/rock interactions during contact metamorphism and anatexis, Lithos, 125, 503–520, https://doi.org/10.1016/j.lithos.2011.03.007, 2011.
Castor, S. B.: The Mountain Pass rare-earth carbonatite and associated ultrapotassic rocks, California, The Canadian Mineralogist, 46, 779–806, https://doi.org/10.3749/canmin.46.4.779, 2008.
Chakhmouradian, A. R. and Wall, F.: Rare Earth Elements: Minerals, Mines, Magnets (and More), Elements, 8, 333–340, https://doi.org/10.2113/gselements.8.5.333, 2012.
Chao, E. C. T., Back, J. M., Minkin, J. A., Tatsumoto, M., Wang, J., Conrad, J. E, McKee, E. H., Hou, Z. L., Meng, Q. R., and Huang, S. G.: The sedimentary carbonate-hosted giant Bayan Obo REE-Fe-Nb ore deposit of Inner Mongolia, China: a corner stone example for giant polymetallic ore deposits of hydrothermal origin, USGS Bulletin, 2143, 1–65, https://doi.org/10.3133/b2143, 1997.
Chandler, R., Bhat, G., Mavrogenes, J., Knell, B., David, R., and Leggo, T.: The primary geology of the Paleoproterozoic Mt Weld carbonatite complex, Western Australia, J. Petrol., 65, egae007, https://doi.org/10.1093/petrology/egae007, 2024.
Chebotarev, D. A., Doroshkevich, A., Klemd, R., and Karmanov, N.: Evolution of Nb-mineralization in the Chuktukon carbonatite massif, Chadobets upland (Krasnoyarsk Territory, Russia), Period. Mineral., 86, 99–118, https://doi.org/10.2451/2017PM733, 2017.
Chen, W., Honghui, H., Bai, T., and Jiang, S.: Geochemistry of Monazite within Carbonatite Related REE Deposits, Resources, 6, 51, https://doi.org/10.3390/resources6040051, 2017.
Chiquet, A., Michard, A., Nahon, D., and Hamelin, B.: Atmospheric input vs in situ weathering in the genesis of calcretes: an Sr isotope study at Gálvez (Central Spain), Geochim. Cosmochim. Ac., 63, 311–323, https://doi.org/10.1016/S0016-7037(98)00271-3, 1999.
Christy, A. G. and Atencio, D.: Clarification of status of species in the pyrochlore supergroup, Mineral. Mag., 77, 13–20, https://doi.org/10.1180/minmag.2013.077.1.02, 2013.
Christy, A. G., Pekov, I. V., and Krivovichev, S. G.: The Distinctive Mineralogy of Carbonatites, Elements, 17, 333–338, https://doi.org/10.2138/gselements.17.5.333, 2021.
Coello, J., Cantagrel, J. M., Hernán, F., Fúster, J. M., Ibarrola, E., Ancochea, E., Casquet, C., Jamond, C., Díaz-de-Terán, J. R., and Cendrero, A.: Evolution of the Eastern volcanic ridge of Canary Islands based on new K-Ar data, J. Volcanol. Geoth. Res., 53, 251–274, https://doi.org/10.1016/0377-0273(92)90085-R, 1992.
Connelly, N. G., Hartshorn, R. M., Damhus, T., and Hutton, A. T.: Nomenclature of Inorganic Chemistry IUPAC Recommendations 2005, RSC Publishing, Cambridge, ISBN-0-85404-438-8, 2005.
Courtillot, V., Davaille, A., Besse, J., and Stock, J.: Three distinct types of hotspots in the Earth's mantle, Earth Planet. Sc. Lett., 205, 295–308, https://doi.org/10.1016/S0012-821X(02)01048-8, 2003.
De Ignacio, C., Muñoz, M., and Sagredo, J.: Carbonatites and associated nephelinites from São Vicente, Cape Verde Islands, Mineral. Mag., 76, 311–355, https://doi.org/10.1180/minmag.2012.076.2.05, 2012.
Demény, A., Ahijado, A., Casillas, R., and Vennemann, T. W.: Crustal contamination and fluid/rock interaction in the carbonatites of Fuerteventura (Canary Islands, Spain): A C, O, H isotope study, Lithos, 44, 101–115, https://doi.org/10.1016/S0024-4937(98)00050-4, 1998.
Dostal, J.: Rare Earth Element Deposits of Alkaline Igneous Rocks, Resources, 6, 34–46, https://doi.org/10.3390/resources6030034, 2017.
Doucelance, R., Hammouda, T., Moreira, M., and Martins, J. C.: Geochemical constraints on depth of origin of oceanic carbonatites: The Cape Verde case, Geochim. Cosmochim. Ac., 74, 7261–7282, https://doi.org/10.1016/j.gca.2010.09.024, 2010.
Doucelance, R., Bellot, N., Boyet, M., Hammouda, T., and Bosq, C.: What coupled cerium and neodymium isotopes tell us about the deep source of oceanic carbonatites, Earth Planet. Sc. Lett., 407, 175–186, https://doi.org/10.1016/j.epsl.2014.09.042, 2014.
European Commission: European Green Deal, https://commission.europa.eu/strategy-and-policy/priorities-2019-2024/european-green-deal_en (last access: 10 April 2024), 2019.
European Commission: Study on the Critical Raw Materials for the EU 2023 – Final Report, https://op.europa.eu/en/publication-detail/-/publication/57318397-fdd4-11ed-a05c-01aa75ed71a1 (last access: 26 May 2023), 2023a.
European Commission: Regulation of the European Parliament and of the Council establishing a framework for ensuring a secure and sustainable supply of critical raw materials and amending Regulations (EU) 168/2013, (EU) 2018/858, 2018/1724 and (EU) 2019/1020, https://eur-lex.europa.eu/legal-content/EN/TXT/?uri=CELEX%3A52023PC0160 (last access: 16 March 2023), 2023b.
FAO/UNESCO: Soil Map of the World Project 1:5 000 000, Chart VI1, https://www.fao.org/soils-portal/data-hub/soil-maps-and-databases/faounesco-soil-map-of-the-world/en/ (last access: 1 January 2024), 1974.
Feraud, G., Giannerini, G., Campredon, R., and Stillman, C. J.: Geochronology of some canarian dike swarms: contribution to the volcano-tectonic evolution of the archipielago, J. Volcanol. Geoth. Res., 25, 29–52, https://doi.org/10.1016/0377-0273(85)90003-4, 1985.
Fernández, C., Casillas, R., Ahijado, A., Perelló, V., and Hernández-Pacheco, A.: Shear zones as a result of intraplate tectonics in oceanic crust: the example of the Basal Complex of Fuerteventura (Canary Islands), J. Struct. Geol., 19, 41–57, https://doi.org/10.1016/S0191-8141(96)00074-0, 1997.
Frisch, T., Schmincke, H. U., and Sumita, M.: Geological evolution of the Canary Islands: a young volcanic archipelago adjacent to the old African continent, B. Volcanol., 74, 1255–1256, https://doi.org/10.1007/s00445-012-0605-1, 2012.
Fúster, J. M., Cendrero, A., Gastesi, P., Ibarrola, E., and López-Ruiz, J.: Geología y volcanología de las Islas Canarias – Fuerteventura, Instituto “Lucas Mallada”, Consejo Superior de Investigaciones Científicas, Madrid, 239 pp., 1968.
Goodenough, K. M., Schilling, J., Jonsson, E., Kalvig, P., Charles, N., Tuduri, J., Deady, E. A., Sadeghi, M., Schiellerup, H., Müller, A., Bertrand, G., Arvanitidis, N., Eliopoulos, D. G., Shaw, R. A., Thrane, K., and Keulen, N.: Europe's rare earth element resource potential: An overview of REE metallogenetic provinces and their geodynamic setting, Ore Geol. Rev., 72, 838–856, https://doi.org/10.1016/j.oregeorev.2015.09.019, 2016.
Goudie, A. S. and Middleton, N. J.: Saharan dust storms: nature and consequences, Earth Sci. Rev., 56, 179–204, https://doi.org/10.1016/S0012-8252(01)00067-8, 2001.
Gutiérrez, M., Casillas, R., Fernández, C., Balogh, K., Ahijado, A., Castillo, C., Colmenero, J. R., and García-Navarro, E.: The submarine volcanic succession of the basal complex of Fuerteventura, Canary Islands: A model of submarine growth and emergence of tectonic volcanic islands, B. Geol. Soc. Am., 118, 785–804, https://doi.org/10.1130/B25821.1, 2006.
Gutiérrez-Elorza, M., Lucha, P., Gracia, F. J., Desir, G., Marín, C., and Petit-Maire, N.: Palaeoclimatic considerations of talus flatirons and aeolian deposits in Northern Fuerteventura volcanic island (Canary Islands, Spain), Geomorphology, 197, 1–9, https://doi.org/10.1016/j.geomorph.2011.09.020, 2013.
Haxel, G. B.: Ultrapotassic mafic dikes and rare earth element- and barium-rich carbonatite at Mountain Pass, Mojave Desert, southern California: summary and field trip localities, U.S. Geol. Surv. Open-File Rep., 1219, http://pubs.usgs.gov/of/2005/1219/ (last access: 20 March 2006), 2005.
Hobson, A., Bussy, F., and Hernández, J.: Shallow-level migmatization of gabbrosin a metamorphic contact aureole, Fuerteventura Basal Complex, Canary Islands, J. Petrol., 39, 1025–1037, https://doi.org/10.1093/petroj/39.5.1025, 1998.
Hoernle, K., Tilton, G., Le Bas, M. J., Duggen, S., and Garbe-Schönberg, D.: Geochemistry of oceanic carbonatites compared with continental carbonatites: Mantle recycling of oceanic crustal carbonate, Contrib. Mineral. Petr., 142, 520–542, https://doi.org/10.1007/s004100100308, 2002.
Holloway, M. I. and Bussy, F.: Trace element distribution among rock-forming minerals from metamorphosed to partially molten basic igneous rocks in a contact aureole (Fuerteventura, Canaries), Lithos, 102, 616–639, https://doi.org/10.1016/j.lithos.2007.07.026, 2008.
Huerta, P., Rodríguez-Berriguete, A., Martín-García, R., Martín-Pérez, A., La-Iglesia-Fernández, A., and Alonso-Zarza, A.: The role of climate and eolian dust input in calcrete formation in volcanic islands (Lanzarote and Fuerteventura, Spain), Palaeogeogr. Palaeocl., 417, 66–79, https://doi.org/10.1016/j.palaeo.2014.10.008, 2015.
Humphreys-Williams, E. R. and Zahirovic, S.: Carbonatites and Global Tectonics, Elements, 17, 339–344, https://doi.org/10.2138/gselements.17.5.339, 2021.
Jahn, R., Blume, H. P., Asio, V. B., Spaargaren, O., and Schad, P.: Guidelines for soil description, FAO, Rome, 97 pp., https://www.fao.org/3/a0541e/a0541e.pdf (last access: 1 January 2006), 2006.
Kamenetsky, V. S., Doroshkevich, A. G., Elliot, H. A. L., and Zaitsev, A. N.: Carbonatites: Contrasting, Complex, and Controversial, Elements, 17, 307–314, https://doi.org/10.2138/gselements.17.5.307, 2021.
Kravchenko, S. M. and Pokrovsky, B. G.: The Tomtor alkaline ultrabasic massif and related REE-Nb deposits, northern Siberia, Econ. Geol., 90, 676–689, https://doi.org/10.2113/gsecongeo.90.3.676, 1995.
Kravchenko, S. M., Czamanske, G., and Fedorenko, V. A.: Geochemistry of carbonatites of the Tomtor massif, Geochem. Int., 41, 545–558, 2003.
Lai, X., Yang, X., Santosh, M., Liu, Y., and Ling, M.: New data of the Bayan Obo Fe-REE-Nb deposit, Inner Mongolia: Implications for ore gènesis, Precambrian Res., 263, 108–122, https://doi.org/10.1016/j.precamres.2015.03.013, 2015.
Le Bas, M. J.: The pyroxenite-ijolite-carbonatite intrusive igneous complexes of Fuerteventura, Canary Islands, J. Geol. Soc. London, 138, 496, https://doi.org/10.1144/gsjgs.138.4.0493, 1981.
Le Bas, M. J, Rex, D. C., and Stillman, C. J.: The early magmatic chronology of Fuerteventura, Geol. Mag., 123, 287–298, https://doi.org/10.1017/S0016756800034762, 1986.
Le Maitre, R. W., Streckeisen, A., Zanettin, B., Le Bas, M. J., Bonin, B., Bateman, P., Bellieni, G., Dudek, A., Efremova, S., Keller, J., Lameyre, J., Sabine, P. A., Schmid, R., Sorensen, H., and Woolley, A. R.: Igneous Rocks: A Classification and Glossary of Terms, 2nd edn., Cambridge, UK, Cambridge Univ. Press, ISBN 9780521619486, 2005.
Liu, Y. L., Ling, M. X., Williams, I. S., Yang, X. Y., Wang, C. Y., and Sun, W.: The formation of the giant Bayan Obo REE-Nb-Fe deposit, North China, Mesoproterozoic carbonatite overprinted Paleozoic dolomitization, Ore Geol. Rev., 92, 73–83, https://doi.org/10.1016/j.oregeorev.2017.11.011, 2018.
Long, K. R., Van Gosen, B. S., Foley, N. K., and Cordier, D.: The principal rare earth elements deposits of the United States: A summary of domestic deposits and a global perspective, United States Geological Service, https://pubs.usgs.gov/sir/2010/5220/ (last access: 10 January 2013), 2010.
Longpré, M. A. and Felpeto, A.: Historical volcanism in the Canary Islands; part 1: A review of precursory and eruptive activity, eruption parameter estimates, and implications for hazard assessment, J. Volcanol. Geoth. Res., 419, 107363, https://doi.org/10.1016/j.jvolgeores.2021.107363, 2021.
Machado-Yanes, M. C.: Reconstrucción paleoecológica y etnoarqueológica por medio del análisis antracológico. La Cueva de Villaverde, Fuerteventura, in: Biogeografía Pleistocena-Holocena de la Península Ibérica, 261274, edited by: Ramil-Rego, P., Fernández-Rodríguez, C., and Rodríguez-Guitián, M., 261 pp., ISBN 84-453-1716-4, 1996.
Mangas, J., Pérez-Torrado, F. J., Reguillón, R. M., and Cabrera, M. C.: Prospección radiométrica en rocas alcalinas y carbonatitas de la serie plutónica I de Fuerteventura (Islas Canarias). Resultados preliminares e implicaciones metalogénicas, Actas del III Congreso Geológico de España y VIII Congreso Latinoamericano de Geología, Salamanca, 3, 389–393, ISBN 84-600-8114-1, 1992.
Mangas, J., Pérez-Torrado, F. J., Reguillón, R. M., and Martin-Izard, A.: Mineralizaciones de tierras raras ligadas a los complejos intrusivos alcalino-carbonatíticos de Fuerteventura (Islas Canarias), Bol. Soc. Esp. Min., 17, 212–213, 1994.
Mangas, J., Pérez-Torrado, F. J., Reguillón, R. M., and Martin- Izard, A.: Rare earth minerals in carbonatites of Basal Complex of Fuerteventura (Canary Islands, Spain), in: Mineral Deposit: Research and Exploration, where do they meet?, edited by: Papunen, H., Balkema, Rotterdam, 475–478, ISBN 978-9054108894, 1997.
Mariano, A. N. and Mariano Jr., A.: Rare earth mining and exploration in North America, Elements, 8, 369–376, https://doi.org/10.2113/gselements.8.5.369, 2012.
Massari, S. and Ruberti, M.: Rare earth elements as critical raw materials: Focus on international markets and future strategies, Resour. Policy, 38, 36–43, https://doi.org/10.1016/j.resourpol.2012.07.001, 2013.
McDonough, W. and Sun, W.: The composition of the Earth, Chem. Geol., 67, 1050–1056, https://doi.org/10.1016/0009-2541(94)00140-4, 1995.
Méndez-Ramos, J., Acosta-Mora, P., Ruiz-Morales, J. C., Hernández, T., Morge, M. E., and Esparza, P.: Turning into the blue: materials for enhancing TiO2 photocatalysis by up-conversion photonics, RSC Adv., 3, 23028–23034, https://doi.org/10.1039/C3RA44342F, 2013.
Menéndez, I., Díaz-Hernández, J. L., Mangas, J., Alonso, I., and Sánchez-Soto, P. J.: Airborne dust accumulation and soil development in the North-East sector of Gran Canaria (Canary Islands, Spain), J. Arid Environ., 71, 57–81, https://doi.org/10.1016/j.jaridenv.2007.03.011, 2007.
Menéndez, I., Campeny, M., Quevedo-González, L., Mangas, J., Llovet, X., Tauler, E., Barrón, V., Torrent, J., and Méndez-Ramos, J.: Distribution of REE-bearing minerals in felsic magmatic rocks and paleosols from Gran Canaria, Spain: Intraplate oceanic islands as a new example of potential, non-conventional sources of rare-earth elements, J. Geochem. Explor., 204, 270–288, https://doi.org/10.1016/j.gexplo.2019.06.007, 2019.
Moore, M., Chakhmouradian, A., Mariano, A. N., and Sidhu, R.: Evolution of Rare-earth Mineralization in the Bear Lodge Carbonatite, Ore Geol. Rev., 64, 499–521, https://doi.org/10.1016/j.oregeorev.2014.03.015, 2015.
Mourão, C., Mata, J., Doucelance, R., Madeira, J., da Silveira, A. B., Silva, L. C., and Moreira, M.: Quaternary extrusive calciocarbonatite volcanism on Brava Island (Cape Verde): A nephelinite-carbonatite immiscibility product, J. Afr. Earth Sci., 56, 59–74, https://doi.org/10.1016/j.jafrearsci.2009.06.003, 2010.
Muñoz, M.: Ring complexes of Pájara in Fuerteventura Island, Bulletin Volcanologique, 33, 840–861, https://doi.org/10.1007/BF02596753, 1969.
Muñoz, M., Sagredo, J., de Ignacio, C., Fernández-Suárez, J., and Jeffries, T. E.: New data (U-Pb, K-Ar) on the geochronology of the alkaline-carbonatitic association of Fuerteventura, Canary Islands, Spain, Lithos, 85, 140–153, https://doi.org/10.1016/j.lithos.2005.03.024, 2005.
Olson, J. C., Shawe, D. R., Pray, L. C., and Sharp, W. N.: Rare-Earth Mineral Deposits of the Mountain Pass District, San Bernardino County, California, Science, 119, 325–326, https://doi.org/10.1126/science.119.3088.325, 1954.
Park, J. and Rye, D. M.: Broader Impacts of the Metasomatic Underplating Hypothesis, Geochem. Geophy. Geosy., 20, 4180–4829, https://doi.org/10.1029/2019GC008493, 2019.
Pérez-Torrado, F. J., Carracedo, J. C., Guillou, H., Rodríguez-González, A., and Fernández-Turiel, J. L.: Age, duration, and spatial distribution of ocean shields and rejuvenated volcanism: Fuerteventura and Lanzarote, Eastern Canaries, J. Geol. Soc. London, 180, jgs2022-112, https://doi.org/10.1144/jgs2022-112, 2023.
Pirajno, F. and Yu, H. C.: The carbonatite story once more and associated REE mineral systems, Gondwana Res., 107, 281–295, https://doi.org/10.1016/j.gr.2022.03.006, 2022.
Reinhardt, N., Proenza, J., Villanova-de-Benavent, C., Aiglsperger, T., Bover-Arnal, T., Torró, L., Salas, R., and Dziggel, A.: Geochemistry and Mineralogy of Rare Earth Elements (REE) in Bauxitic Ores of the Catalan Coastal Range, NE Spain, Minerals, 8, 562, https://doi.org/10.3390/min8120562, 2018.
Rudnick, R. L. and Gao, S.: Composition of the Continental Crust, in: Treatise on Geochemistry, edited by: Holland, H. H. and Turekian, K. K., 4, 1–51, https://doi.org/10.1016/B978-0-08-095975-7.00301-6, 2014.
Scheuvens, D., Schütz, L., Kandler, K., Ebert, M., and Weinbruch, S.: Bulk composition of northern African dust and its source sediments – a compilation, Earth Sci. Rev., 116, 170–194, https://doi.org/10.1016/j.earscirev.2012.08.005, 2013.
Schmincke, H. and Sumita, M.: Geological evolution of the Canary Islands: a young volcanic archipelago adjacent to the old African Continent, edited by: Németh, K., Koblenz, 200 pp., ISBN 978-3-86972-005-0, 2010.
Smith, M. P., Campbell, L. S., and Kynicky, J.: A review of the genesis of the world class Bayan Obo Fe-REE-Nb deposits, Inner Mongolia, China: multistage processes and outstanding qüestions, Ore Geol. Rev., 64, 459–476, https://doi.org/10.1016/j.oregeorev.2014.03.007, 2015.
Smith, M. P., Moore, K., Kavecsánszki, D., Finch, A. A., Kynicky, J., and Wall, F.: From mantle to critical zone: A review of large and giant-sized deposits of the rare earth elements, Geosci. Front., 7, 315–334, https://doi.org/10.1016/j.gsf.2015.12.006, 2016.
Steiner, C., Hobson, A., Favre, P., and Stampli, G. M.: Early Jurassic sea-floor spreading in the central Atlantic – the Jurassic sequence of Fuerteventura (Canary Islands), Geol. Soc. Am. Bull., 110, 1304–1317, https://doi.org/10.1130/0016-7606(1998)110<1304:MSOFCI>2.3.CO;2, 1998.
Torró, L., Villanova, C., Castillo, M., Campeny, M., Gonçalves, A. O., and Melgarejo, J. C.: Niobium and rare earth minerals from the Virulundo carbonatite, Namibe, Angola, Mineral. Mag., 76, 393–409, https://doi.org/10.1180/minmag.2012.076.2.08, 2012.
Torró, L., Proenza, J. A., Aiglsperger, T., Bover-Arnal, T., Villanova-de-Benavent, C., Rodríguez-García, D., Ramírez, A., Rodríguez, J., Mosquea, L. A., and Salas, R.: Geological, geochemical and mineralogical characteristics of REE-bearing Las Mercedes bauxite deposit, Dominican Republic, Ore Geol. Rev., 89, 114–131, https://doi.org/10.1016/j.oregeorev.2017.06.017, 2017.
Troll, V. and Carracedo, J. C.: The Geology of Fuerteventura, in: The Geology of Canary Islands, edited by: Troll, V., Carracedo, J. C., and Weismaier, S., Elsevier, 531–582, https://doi.org/10.1016/B978-0-12-809663-5.00008-6, 2016.
van den Bogaard, P.: The origin of the Canary Island Seamount Province – New ages of old seamounts, Sci. Rep., 3, 2107, https://doi.org/10.1038/srep02107, 2013.
Wang, Q., Deng, J., Liu, X., Zhang, Q., Sun, S., Jiang, C., and Zhou, F.: Discovery of the REE minerals and its geological significance in the Quyang bauxite deposit, West Guangxi, China, J. Asian Earth Sci., 39, 701–712, https://doi.org/10.1016/j.jseaes.2010.05.005, 2010.
Wang, X., Jiao, Y., Du, Y., Ling, W., Wu, L., Cui, T., Zhou, Q., Jin, Z., Lei, Z., and Wen, S.: REE mobility and Ce anomaly in bauxite deposit of WZD area, Northern Guizhou, China, J. Geochem Explor., 133, 103–117, https://doi.org/10.1016/j.gexplo.2013.08.009, 2013.
Warr, L. N.: IMA–CNMNC approved mineral symbols, Mineral. Mag., 85, 291–320, https://doi.org/10.1180/mgm.2021.43, 2021.
Weidendorfer, D., Schmidt, M. W., and Mattsson, H. B.: Fractional crystallization of Si-undersaturated alkaline magmas leading to unmixing of carbonatites on Brava Island (Cape Verde) and a general model of carbonatite genesis in alkaline magma suites, Contrib. Miner. Petr., 171, 1–29, https://doi.org/10.1007/s00410-016-1249-5, 2016.
Wondraczek, L., Tyystjärvi, E., Méndez-Ramos, J., Müller, F. A., and Zhang, Q.: Shifting the Sun: Solar Spectral Conversion and Extrinsic Sensitization in Natural and Artificial Photosynthesis, Adv. Sci., 2, 1500218, https://doi.org/10.1002/advs.201500218, 2015.
Wu, C.: Bayan Obo Controversy: Carbonatites versus Iron Oxide-Cu-Au-(REE-U), Resour. Geol., 58, 348–354, https://doi.org/10.1111/j.1751-3928.2008.00069.x, 2008.
Yang, K., Fan, H., Pirajno, F., and Li, X.: The bayan Obo (China) giant REE accumulation conundrum elucidated by intense magmatic differentiation of carbonatite, Geology, 47, 1198–1202, https://doi.org/10.1130/G46674.1, 2019.
Yaxley, G. M., Anenburg, M., Tappe, S., Decree, S., and Guzmics, T.: Carbonatites: Classification, Sources, Evolution, and Emplacement, Annual Reviews on Earth and Planetary Sciences, 50, 261–293, https://doi.org/10.1146/annurev-earth-032320-104243, 2022.
Zazo, C., Goy, J. L., Hillaire-Marcel, C., Gillot, P. Y., Soler, V., González, J. A., Dabrio, C. J., and Ghaleb, B.: Raised marine sequences of Lanzarote and Fuerteventura revisited – a reappraisal of relative sea-level changes and vertical movements in the eastern Canary Islands during the Quaternary, Quaternary Sci. Rev., 21, 2019–2046, https://doi.org/10.1016/S0277-3791(02)00009-4, 2002.
Zhukova, I. A., Stepanov, A. S., Jiang, S. Y., Murphy, D., Mavrogenes, J., Allen, C., Chen, W., and Bottrill, R.: Complex REE systematics of carbonatites and weathering products from uniquely rich Mount Weld REE deposit, Western Australia, Ore Geol. Rev., 139, 104539, https://doi.org/10.1016/j.oregeorev.2021.104539,2021.