the Creative Commons Attribution 4.0 License.
the Creative Commons Attribution 4.0 License.
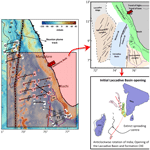
Cretaceous–Paleocene extension at the southwestern continental margin of India and opening of the Laccadive basin: constraints from geophysical data
Mathews George Gilbert
Parakkal Unnikrishnan
Munukutla Radhakrishna
Previous geophysical investigations of the western continental margin of India (WCMI) confirm the two-phase breakup history of the margin with the first breakup taking place between India and Madagascar that created the Mascarene Basin in the Late Cretaceous and the second breakup event in Early Paleocene with Seychelles separating from India. Despite numerous geoscientific studies along the WCMI, the opening of the Laccadive basin, situated along the southern part of the margin, remains poorly constrained. In this study, we evaluate the multi-channel seismic reflection and gravity anomalies at the margin to identify the early rift signatures in conjunction with the magnetic anomaly identifications in the Mascarene Basin. The analysis led to the identification of two trends of extensional structures, a NNW–SSE-oriented structure over the Laccadive Ridge north of Tellicherry Arch, interpreted to result from ENE–WSW extension, and a SSW–NNE-oriented structure in the Laccadive basin region towards the south, interpreted to result from NW–SE extension. Previous plate reconstruction models of the Mascarene Basin using marine magnetic lineations suggest that the ENE–WSW extension observed over the Laccadive Ridge could be related to the India–Madagascar separation. We associate the pattern of sediment deposition and the presence of a Paleocene trap volcanics, linked with the NW–SE grabens observed in the Laccadive basin region, to the extension between the Laccadive Ridge and the western coast of India after the separation of Madagascar from India. We further propose that the anticlockwise rotation of India and the passage of the Réunion plume have facilitated the opening of the Laccadive basin.
- Article
(10195 KB) - Full-text XML
-
Supplement
(3230 KB) - BibTeX
- EndNote
The breakup of Gondwanaland into eastern and western Gondwana during the Early Jurassic period initiated the formation of the western Indian Ocean (Fig. 1a–b). The subsequent breakup of Madagascar and the Seychelles from India, and the seafloor spreading along the Carlsberg and Central Indian ridges culminated in the development of the present-day northwest Indian Ocean. The Laccadive Ridge, the Maldives Ridge, the Chagos Bank, the Saya de Malha Bank, the Nazareth Bank, Mauritius, and Réunion are the major topographic features present in the northwestern Indian Ocean (Fig. 1a), and some of these are believed to be micro-continents (Torsvik et al., 2013).
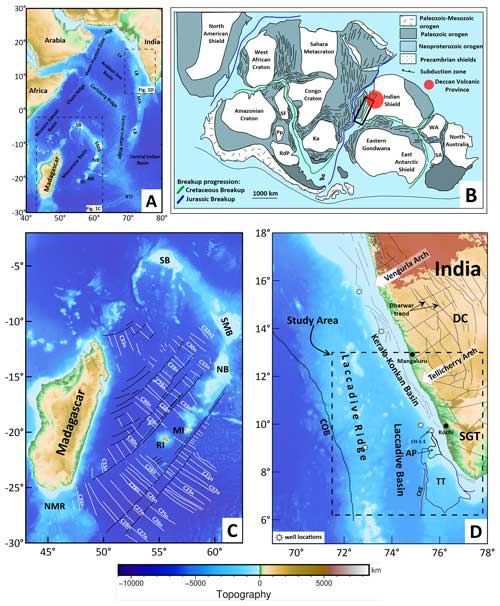
Figure 1(a) Regional tectonic map of the northwestern Indian Ocean with satellite-derived seafloor topography (Smith and Sandwell, 1997). (b) Map showing the position of India relative to Madagascar and the Deccan Volcanic Province in its late Paleozoic fit (Lovecchio et al., 2020, modified after). The area of interest is marked in black rectangle. (c) Tectonic map of Madagascar and the Mascarene Basin showing mapped seafloor-spreading-type magnetic lineations in solid white lines (Bhattacharya and Yatheesh, 2015; Shuhail et al., 2018, and references therein). Solid black lines represent the mapped fracture zones or pseudo-faults. (d) Regional tectonic map of the western continental margin of India (Smith and Sandwell, 1997). Solid black lines in the offshore region represent shear zones and faults. The location of the maps in panels (c) and (d) are shown in panel (a). SB stands for Seychelles Bank, SMB stands for Saya de Malha Bank, NB stands for Nazareth Bank, MI is Mauritius, RI is Réunion, RTJ stands for Rodrigues Triple Junction, LR stands for Laccadive Ridge, MA is the Maldives, CB stands for Chagos Bank, MR stands for Murray Ridge, Lx is Laxmi Ridge, RdP stands for Río de la Plata, Ka is the Kalahari, Pp stands for Paraná Panema, SF stands for São Francisco, WA stands for western Australia, SA stands for southern Australia, NMR stands for northern Madagascar Ridge, DC stands for Dharwar Craton, SGT stands for Southern Granulite Terrane, LB stands for Laccadive basin, AP stands for Alleppey Platform, TT stands for Trivandrum Terrace, CKE stands for Chain-Kairali Escarpment, and COB stands for continent–ocean boundary.
The western continental margin of India (WCMI) formed through the breakup and separation of India and Madagascar in the Late Cretaceous (Storey et al., 1995; Pande et al., 2001). This breakup event resulted in the formation of the Mascarene Basin (Fig. 1a and c), the details of which are recorded in the magnetic anomalies in the basin (Fig. 1c). The northern part of the margin then experienced another breakup event when the Seychelles block separated from the combined Laxmi Ridge and India in the Early Paleocene time. This second breakup event is well studied with the pre-drift juxtaposition of the continental blocks fairly well established from the magnetic anomaly identifications and geochronology (Collier et al., 2008; Chaubey et al., 2002; Ganerød et al., 2011; Shellnutt et al., 2015, 2017). The southern part of the margin is considered to be conjugate with the eastern Madagascar margin (Katz and Premoli, 1979) based on the continuity of the major shear zones and coastlines matched at 1000 m isobath. However, recent close-fit reconstruction models have incorporated the continental fragments like the Laccadive Ridge (Bhattacharya and Yatheesh, 2015) or Mauritia (comprised of Mauritius, the southern Mascarene Plateau, the Laccadive Ridge, and the Chagos Bank) (Torsvik et al., 2013) between India and Madagascar in the India–Madagascar pre-drift scenario and suggested a breakup timing of around 83 Ma.
The Laccadive Ridge is the bathymetric high feature in the southwest offshore margin and lies parallel to the western coast of India (Fig. 1d). The Laccadive basin lies between the Laccadive Ridge and the continental shelf south of Tellicherry Arch (Fig. 1d). The southwestern margin of India was affected by Réunion plume volcanism towards the end of the Cretaceous (Singh and Lal, 1993) as revealed by the presence of a widespread layer of trap volcanics below the Tertiary sediment cover at the margin (Singh et al., 2007; Singh and Lal, 1993).
Most of the drilled wells along this margin were terminated in the Late Cretaceous to Early Paleocene trap layer and at present none have encountered the crystalline basement. By contrast, the CH-1-1 well located within the shelf (Fig. 1d) penetrated through the Paleocene trap, encountered Santonian formations, and terminated in the Late Cretaceous felsic volcanics (Singh and Lal, 1993). One of the key questions that has not been resolved concerns the absence of Late Cretaceous sediments in the Laccadive basin as a whole: what caused this more than 20 Myr gap in the sedimentary record between the India–Madagascar breakup at 83 Ma and the oldest Paleogene sediments? This long time gap indicates either the presence of older sediments below the Paleocene trap layer or the opening of the Laccadive basin subsequent to India–Madagascar separation. Further, the presence of the continental fragments between India and Madagascar (Bhattacharya and Yatheesh, 2015; Torsvik et al., 2013) makes for a complex geodynamic setting, considering how this separation took place, and therefore it provides some insights into the impact of pre-existing lithospheric inheritance. Hence, examining the development of the Laccadive basin will provide important constraints on the early breakup evolution of the WCMI.
In this study, we made a correlative analysis of the multi-channel seismic data with the residual gravity anomalies, which provided evidence of a major extensional event that occurred at the southwestern part of the margin that is not related to the India–Madagascar breakup. The time stamping of this major extensional event provides important constraints on the evolution of the WCMI and helps to build improved plate tectonic reconstruction models.
The study area lies south of Tellicherry Arch and contains the southern part of the Laccadive Ridge and the Laccadive basin (Fig. 1d). The major geomorphic features present in the study area from west to east are the Laccadive Ridge, the Laccadive basin, the Alleppey Platform and Trivandrum Terrace (together called the Alleppey–Trivandrum Terrace Complex), and the continental shelf (Fig. 1d). The Alleppey–Trivandrum Terrace Complex is characterised by horst–graben structures and bounded in the west by the Chain-Kairali Escarpment (CKE) feature (Yatheesh et al., 2006, 2013; Nathaniel, 2013) as revealed in seismic sections (See Fig. S1 in the Supplement). Numerous seamounts, guyots, and knolls are present in the region between the Laccadive Ridge and the continental shelf within the Laccadive basin (See Fig. S2) (Bijesh et al., 2018). In addition, the entire region is characterised by several intrusive structures within the tertiary sediments (Unnikrishnan et al., 2023). The southern part of the Cannanore Rift System (CRS) identified over the Laccadive Ridge (Fig. 2) from the Director General of Hydrocarbon (, ) lies within the study area.
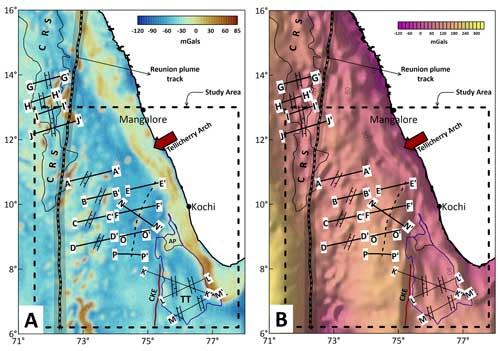
Figure 2(a) Satellite-derived free-air gravity anomaly map (Sandwell et al., 2014). (b) Crustal Bouguer anomaly map. The location and orientation of identified extensional features, grabens, and intrusives are marked. Black solid lines represent the location of the profiles. Interpreted seismic sections are shown in Fig. 5. The thick broken black line in the centre of the basin represents the identified volcanic ridge. (refer to Figs. 5 and S1 for seismic sections). The location of the maps is shown in Fig. 1a. CRS represents the Cannanore Rift System as identified by (). CKE stands for Chain-Kairali Escarpment, AP stands for Alleppey Platform, and TT stands for Trivandrum Terrace.
In this study, we used the satellite-derived free-air gravity (Fig. 2a) (Sandwell et al., 2014) and bathymetry from the General Bathymetric Chart of the Oceans (Fig. 1d) (GEBCO, 2020) for comparative analysis with multi-channel seismic reflection profiles (Figs. 2b–5). The large volume of industry seismic reflection data (see Fig. S3) at this margin provided information on the sediment thickness above the Paleocene trap layer and various horizons within the post-Paleocene sediments (Unnikrishnan et al., 2023). We also gathered a few published seismic sections (Nathaniel, 2013; Yatheesh et al., 2013) within the study area (see Fig. S1).
The gravity effects of the water layer and the sediments are removed from the satellite-derived free-air anomaly data to obtain crustal Bouguer anomalies. High-resolution sediment thickness derived from two-way travel time (TWT) maps is used to calculate the gravity effect of the sediments. The TWT maps are available for three different times: the Early Paleocene, Early Eocene, Early Miocene (Unnikrishnan et al., 2023). These maps were converted to depth with respective interval velocities (after Unnikrishnan et al., 2023) and the total sediment layer is used to calculate the gravity effect of sediments. A density of 2300 kg m−3 for sediments and 1030 kg m−3 for the water column is used. For the crustal rocks, an average density of 2800 kg m−3 was considered as the study area lies within the extended continental crust (Unnikrishnan et al., 2023). A 10–200 km wavelength band-pass filter was applied to the crustal Bouguer anomaly map to highlight the crustal heterogeneities, and the first vertical derivative (FVD) and the total horizontal derivative (THD) of the band-pass-filtered map were prepared to identify shallow structural features. The location of the identified features such as rifts and volcanic intrusives in the seismic sections are then transferred on to these gravity anomaly maps in order to understand their continuity. Subsequently, the sediment isochron maps were interpreted to understand the timing of opening of the Laccadive basin with inputs from interpreted seismic sections. Scientifically derived colour maps are used to prepare maps (Crameri et al., 2020).
4.1 Sediment isochron map analysis
The isochron maps in the study area (Fig. 3) show that for the Early Paleocene to Early Eocene time interval, significant sediment deposition occurred parallel to the coast with much less sedimentation in the Laccadive basin. During this period, maximum deposition took place in the area between Tellicherry Arch and off Kochi (the marked sediment patch in Fig. 3a) with a minor sediment channel extending into the basin (the marked sediment channel in Fig. 5a). During the Early Eocene to Early Miocene time interval, deposition within the sediment patch was almost absent, whereas, significant deposition is observed in the northern part of the Laccadive basin on either side of the identified volcanic intrusives (discussed below) (Fig. 5b). From Early Miocene to recent times, sedimentation has been uniform in the Laccadive basin (Fig. 3c). Sediment deposition along the coast remained high throughout the time intervals (Fig. 3a–c). The total sediment deposition pattern shows that most of the sediments were accommodated parallel to the coast and towards the south there is an axis of high sediment deposition into the Laccadive basin (Fig. 3d).
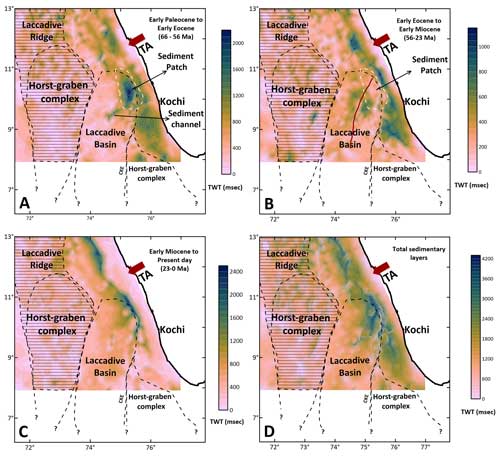
Figure 3Isochron maps from Unnikrishnan et al. (2023) for selected time intervals: (a) Early Paleocene to Early Eocene, (b) Early Eocene to Early Miocene, (c) Early Miocene to Present day, and (d) total sedimentary layers. The brown line in panel B represents trend of the identified volcanic ridge. (Refer to text for detailed explanation and interpretation). TA stands for Tellicherry Arch, while CKE stands for Chain-Kairali Escarpment.
4.2 Gravity anomaly mapping
The crustal Bouguer anomaly map reveal two structural trends: NNW–SSE orientation in the area north of the Tellicherry Arch and NNE–SSW orientation south of Tellicherry Arch (see Fig. 2b). The band-pass-filtered map of the crustal Bouguer anomaly and its first vertical derivative and total horizontal derivative maps in the study area further enhanced the structural features observed in the crustal Bouguer anomaly map (Fig. 4).
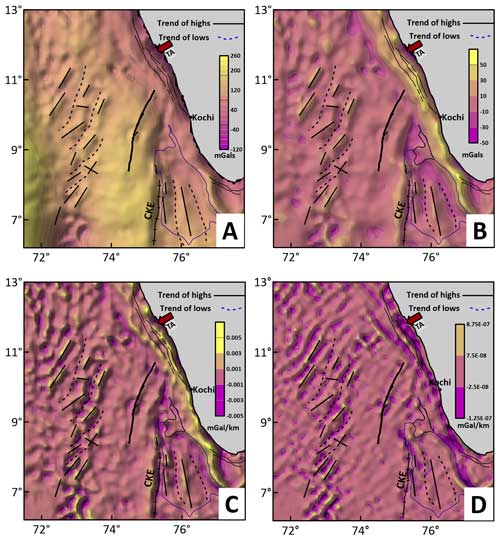
Figure 4(a) The crustal Bouguer anomaly, (b) the band-pass-filtered crustal Bouguer anomaly, (c) the first vertical derivative (FVD) of the band-pass-filtered crustal Bouguer anomaly, and (d) the total horizontal derivative (THD) of the band-pass-filtered crustal Bouguer anomaly. The black lines show the structural highs, and the dotted black lines show the continuity of rift basins identified. The solid and ticked black line represents the Chain-Kairali Escarpment (CKE). The thick solid black line along the centre of the basin represents the identified volcanic ridge. The thin black lines close to the coast represent major faults identified on the continental shelf Singh and Lal (1993). TA stands for Tellicherry Arch.
4.3 Seismic interpretation
We present 13 interpreted seismic sections (Fig. 5) that reveal several rift-related horst–graben structures (extensional features) at the margin. We correlated these structures with the gravity anomaly trends and noticed that the grabens are oriented NNW–SSE in the area north of the Tellicherry Arch, whereas the grabens are oriented NNE–SSW south of Tellicherry Arch (see Figs. 2, 3, and 5). These identified extensional structures show low gravity anomalies, the continuity of which can be traced as gravity lows surrounded by highs in the anomaly maps (Figs. 2a–b and 4a–d). This is particularly prominent within the study area (south of Tellicherry Arch). The seismic sections also reveal a series of volcanic intrusives in the centre of the Laccadive basin that can also be seen in the gravity anomaly map as a broken chain of highs (Figs. 2, 3 and 4). The NNW–SSE-oriented grabens over the Laccadive Ridge north of Tellicherry Arch are part of the CRS mapped by the DGH.
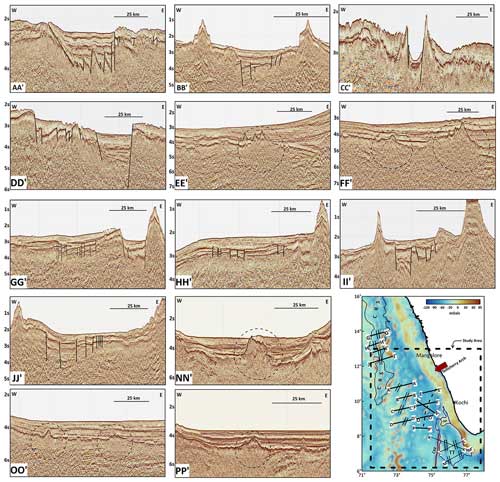
Figure 5Figure showing interpreted seismic sections. The faults are marked, and the intrusives are shown as dotted circles. The inset free-air anomaly map in the bottom right corner shows the location of the seismic profiles. The time is given two-way travel times (TWTs). TWT is the elapsed time for a seismic wave to travel from its source to a given reflector and return to a receiver at the Earth's surface.
This study identifies two major structural trends along the southwestern margin, the first being the NNW–SSE-oriented grabens over the Laccadive Ridge north of Tellicherry Arch, and the second being the NNE–SSW graben system in the Laccadive basin area south of Tellicherry Arch (Fig. 2). The NNW–SSE rifts observed over the Laccadive Ridge conforms with the orientation of the Dharwar structural trend, which is a dominant structural trend in the onshore region north of Tellicherry Arch (Fig. 1d). In addition, these are part of the CRS that is characterised by complex block-like basement structures comprising of grabens, half-grabens, and faults (, ) along the eastern flank of the Laccadive Ridge and extends between 17–9.5° N parallel to the western coast of India (Fig. 2a–b). Previous studies (Kolla and Coumes, 1990; Singh and Lal, 1993) also inferred the continuation of onshore Dharwar trend in the offshore shelf region and beyond. As mentioned earlier, India–Madagascar breakup took place at 83 Ma and resulted in the opening of the Mascarene Basin. Magnetic anomaly identifications together with tectonic-plate reconstruction studies (Shuhail et al., 2018) reveal that during the initial period (83–79 Ma), the spreading in the Mascarene Basin was E–W (Fig. 1c), and this initial spreading regime matches well with the NNW–SSE-oriented rift system observed over the Laccadive Ridge (Fig. 2). Hence, we infer that the NNW–SSE-oriented grabens had formed during the early breakup evolution of the margin between India and Madagascar.
Coming to the margin south of Tellicherry Arch, it is relevant to understand the spreading regime in the Mascarene Basin. Analysis of magnetic anomaly identifications (Bhattacharya and Yatheesh, 2015; Shuhail et al., 2018) reveal that the spreading was more active in the southern part of the basin and also characterised by longer transform faults between the spreading segments. If reconstructed backward to this time (73 Ma reconstruction Shuhail et al., 2018), the identified grabens south of Tellicherry Arch follow the orientation of these transform faults. Tectonic-plate reconstruction efforts by Shuhail et al. (2018) indicate that the spreading in the Mascarene Basin was connected to the study area by long transform faults such as the CKE. Hence, we interpret that the SSW–NNE fault system developed during this time when the study area was in close proximity to the Mascarene Basin spreading centre (Fig. 7, Stage II) due to movement along the transform faults, whereas such large transforms with active spreading were missing in the northern part of the Mascarene Basin, which is conjugate with the Laccadive Ridge region north of Tellicherry Arch. Subsequently, spreading ceased in the Mascarene Basin, and the basin was transferred to the African plate by 57 Ma. Later, these faults opened and extension continued contemporaneous with India's anticlockwise rotation, which facilitated the opening of Laccadive basin as indicated by the sediment isochron maps (Fig. 3a–c).
5.1 Opening of the Laccadive basin
As explained in Sect. 4.1, the sediment deposition in the basin is interpreted from the perspective of the creation of accommodation space and sediment supply. The high-resolution time structure maps (Fig. 3a–d) provide insights into the timing of the opening of the Laccadive basin. These maps clearly reveal significant sediment deposition along the coast-parallel grabens within the shelf part of the margin in all time periods. Further, during the Paleocene–Eocene period, sediment deposition was very significant on the northern fringe of the Laccadive basin (sediment patch in Fig. 3a), with negligible sediments elsewhere in the basin. During the Eocene to Miocene the sediment deposition shifted further offshore into the Laccadive basin (Fig. 3b). The development of the volcanic ridge within the basin also occurred during this time since it is observed that the sediments are deposited on either side of the location of the ridge. Further, the seismic sections show sediments onlapping to the identified volcanic ridge (Fig. 5 sections NN′ and PP′). The comparative analysis of Fig. 3a and b indicates that the basin opened some time after the Early Eocene, and as a result accommodation space was created, with all of the incoming sediments migrating southward into the basin. A small channel of sediment deposition into the Laccadive basin towards the southwest of the sediment patch (marked as sediment channel in Fig. 3a) may represent the initial stage of opening of the basin. During the Miocene to recent period (Fig. 3c), the sediment deposition is more or less uniform throughout the basin. The distal part of the Laccadive basin towards the west has relatively low sediment deposition, which may be due to the area's location far from any sediment supply.
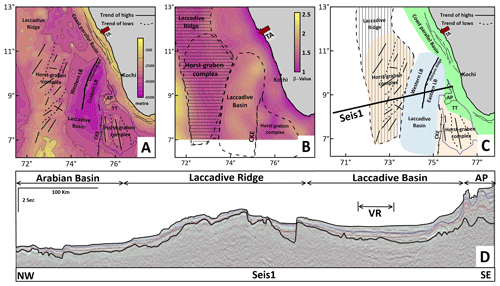
Figure 6(a) Depth to the basement map with all structures identified in the study, (b) β-value map redrawn from Unnikrishnan et al. (2023), (c) proposed tectonic map of the study area. The bottom panel is a seismic section (Seis1) showing the general characteristics of the Laccadive basin area. The seismic section is from Unnikrishnan (2018). The black lines show the structural highs and the dotted black lines show the continuity of rift basins identified. The solid ticked black line represents the Chain-Kairali Escarpment (CKE). The thick solid black line represents the identified volcanic ridge. The thin black lines close to the coast in (a) and (c) represent major faults identified on the continental shelf (Singh and Lal, 1993). TA stands for Tellicherry Arch, AP stands for Alleppey Platform, TT stands for Trivandrum Terrace, VR stands for volcanic ridge, LB stands for Laccadive basin.
Unnikrishnan et al. (2018) identified the Alleppey Platform as a continental fragment and inferred its development during the Oligocene–Miocene period. The Alleppey Platform is located adjacent to the Laccadive basin, and hence the development of the basin and the platform could be related. The timing of the development of the Alleppey platform given by Unnikrishnan et al. (2018) closely agrees with the inferred timing of the opening of the Laccadive basin from this study.
5.2 Distribution of bathymetry highs and intrusives
A striking feature along this margin is the presence of many intrusives and bathymetric highs (see Fig. S2) observed in the seismic and bathymetry data, respectively (Unnikrishnan et al., 2023; Bijesh et al., 2018). These features have very clear expressions on the gravity image of the area (Figs. 2 and 3a–d). The intrusives and bathymetric highs in the study area south of Tellicherry Arch (Fig. S2) appear to be elongated roughly parallel to the trend of the Laccadive basin. In the centre of the Laccadive basin, we noticed a series of volcanic mounds with a trend almost parallel to the CKE (Fig. 2) that are clearly expressed in the seismic sections (Fig. 5). The observed trend correlates well with the crustal Bouguer anomaly map (Fig. 4a) and the depth to basement map prepared by adding the sediment thickness to bathymetry (Fig. 6a). This trend divides the Laccadive basin into eastern and western basins. The composite tectonic map of the study area is shown in Fig. 6c.
Further, the β-value (crustal stretching factor) map (Fig. 6b) calculated by Unnikrishnan et al. (2023) clearly reveals the extensional trend in the study area. The high β values in the centre of the Laccadive basin indicate maximum thinning and conform with our observations in this study.
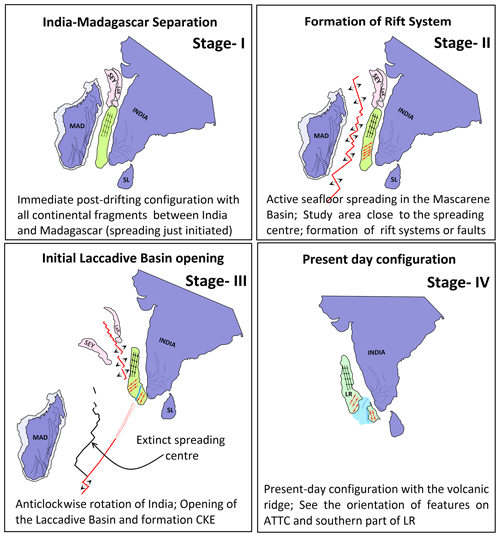
Figure 7Map showing the evolution of the region in four stages. Stage I is the pre-rift juxtaposition of the continental fragments. Note that the Laccadive Ridge is larger since it incorporates all the fragments that are littered between India and Madagascar. Stage II is the formation of the fault systems or the rift systems due to the influence of spreading in the Mascarene Basin. Stage III is the opening of the basin with the CKE, ATTC, and India moving away from the Laccadive Ridge. Note how the orientation of the extensional features changes with the anticlockwise movement of India. Stage IV is the present-day configuration of the margin with all extensional features and the volcanic ridge. LaX is the Laxmi Ridge, SEY is the Seychelles, MAD is Madagascar, SL is Sri Lanka, LR is the Laccadive Ridge, ATTC is the Alleppey–Trivandrum Terrace Complex, and CKE is the Chain-Kairali Escarpment.
5.3 Evolutionary model
Existing plate tectonic reconstruction models (Bhattacharya and Yatheesh, 2015; Shuhail et al., 2018); the pre-existing structural trends along WCMI, India, and Madagascar (Subrahmanyam et al., 1994; Kolla and Coumes, 1990; Bhattacharya and Yatheesh, 2015); and the results from this study have been used to build a schematic evolutionary model for the opening of the Laccadive basin at the southwestern margin of India. Additionally, we also looked at analogue and numerical modelling studies that explain extensional deformation in rifts and rifted margin systems (Péron-Pinvidic and Manatschal, 2010; Zwaan et al., 2021; Bonini et al., 1997; Henza et al., 2010). The locations of India and Madagascar in various stages (I–IV) (Fig. 7) were adopted from the reconstruction model of Shuhail et al. (2018). The salient aspects of each stage are given below.
5.3.1 Stage I
Stage I (Fig. 7, Stage I) shows India and Madagascar along with all the continental fragments in between them and a large number of shear zones onshore for both India and Madagascar (Bhattacharya and Yatheesh, 2015, and references therein) immediately after the spreading started at 83 Ma. These shear zones were used earlier as piercing points to find the relative position of India and Madagascar in the matching of coastlines (Katz and Premoli, 1979; Subrahmanyam and Chand, 2006). Aside from this, studies along the WCMI have shown the extension of onshore structural trends into the offshore region (Subrahmanyam et al., 1994; Kolla and Coumes, 1990) and show that structural inheritance plays a role during rifting and breakup (Péron-Pinvidic and Manatschal, 2010; Zwaan et al., 2021; Bonini et al., 1997; Henza et al., 2010). Dating of volcanics in Madagascar yields an age of 88 Ma (Storey et al., 1995) and magnetic anomalies indicate spreading started around 83 Ma (Shuhail et al., 2018). Hence, we infer that during this extensional span, CRS formed on the Laccadive Ridge following the Precambrian Dharwarian trend that is dominant north of Tellicherry Arch.
5.3.2 Stage II
Stage II (Fig. 7, Stage II) shows the proximity of Laccadive Ridge to the spreading centre in the Mascarene Basin. As discussed in Stage I, the lithosphere between India and Madagascar had zones of weakness (as evidenced by the presence of inherited structures), and as a result, when the area was proximal to the spreading centre, a number of parallel transtensional faults may have formed on the Laccadive Ridge south of Tellicherry Arch. Even though the spreading was happening along the entire basin, the spreading was very active in the southern part of the Mascarene Basin and also characterised by long transform faults (Shuhail et al., 2018). As discussed in Sect. 5.2, distribution of bathymetric highs and intrusives south of Tellicherry Arch provide some evidence for this. It is very likely that later, when the Réunion plume passed over the area, magma may have migrated through the faults formed during this stage, giving rise to the preferred orientation of intrusive and bathymetric features in this area. It is worthwhile to note that Bijesh et al. (2018) related the genesis of the bathymetric features to hotspot volcanism.
5.3.3 Stage III
Stage III (Fig. 7, Stage III) shows the initial stages of opening of the Laccadive basin in the Paleocene. By this time, the entire region was flooded by Deccan volcanics during the passage of Réunion plume. Studies by Patriat and Achache (1984) and Dewey (1989) showed that the Indian plate rotated anticlockwise about 40° since 84 Ma, out of which it underwent about 25° after the soft collision at 50 Ma (Treloar and Coward, 1991). The passage of the Réunion plume over the area may have weakened the overlying lithosphere and, together with India's anticlockwise rotation and the presence of inherited structural weakness, may have led to the reactivation and further extension in the Laccadive basin. The sediment thickness data during the Paleocene–Eocene period shows a sediment channel (Fig. 3a) extending into the Laccadive basin and an area of high sediment deposition (Sediment patch in Fig. 3a), which both indicate the initial stages of opening of the basin. Figure 3b shows sediments being deposited on either side of the volcanic ridge in the Laccadive basin. By this time the basin opened and sediments were accommodated in the basin.
5.3.4 Stage IV
Stage IV (Fig. 7, Stage IV) shows the present-day configuration of the Laccadive basin. The Alleppey–Trivandrum Terrace Complex remains attached to the Indian continent with the CKE forming its western boundary. The centre of the Laccadive basin experienced maximum crustal thinning, and a series of intrusives got emplaced in the crust. The change in the orientation of horst–graben structures in the Alleppey–Trivandrum Terrace Complex from that in the southern part of Laccadive Ridge can be noticed.
The seismic and gravity data analysis along the southern part of the WCMI revealed two significantly different orientations of the extensional graben system, one being NNW–SSE-oriented rifts over the Laccadive Ridge north of Tellicherry Arch, and the other being NNE–SSW-oriented rifts in the Laccadive basin region south of Tellicherry Arch. The change in extensional direction in the Laccadive basin, along with the isochron maps for different times, suggests that the basin opened during the post-Eocene period with maximum extension along the centre of the basin where the volcanic intrusives are emplaced. The lithosphere that existed had zones of weakness, and this along with proximity to the Réunion plume and anticlockwise rotation of India led to complex rifting and evolutionary history for the southern part of the margin.
The authors do not have permission to share data.
The supplement related to this article is available online at: https://doi.org/10.5194/se-15-671-2024-supplement.
MGG: conceptualisation, methodology, validation, formal analysis, writing (original draft and editing), and visualisation. MR: conceptualisation, validation, resources, writing (review and editing), and supervision. PU: conceptualisation and writing (review and editing).
The contact author has declared that none of the authors has any competing interests.
Publisher's note: Copernicus Publications remains neutral with regard to jurisdictional claims made in the text, published maps, institutional affiliations, or any other geographical representation in this paper. While Copernicus Publications makes every effort to include appropriate place names, the final responsibility lies with the authors.
This article is part of the special issue “(D)rifting into the future: the relevance of rifts and divergent margins in the 21st century”. It is not associated with a conference.
We would like to thank the Frank Zwaan for the thorough and constructive review. We sincerely appreciate all valuable comments and suggestions by Yatheesh Vadakkeyakath and the anonymous reviewer, which helped us improve the manuscript. The work benefitted from many constructive discussions with Kondepudi V. S. S. Sai Pattabhiram. This study forms a part of PhD work of Mathews George Gilbert at IIT Bombay. Parakkal Unnikrishnan gratefully acknowledges the permission of the Oil and Natural Gas Corporation Limited (ONGC) provided for the use of the geoscientific data pertaining to the Kerala-Konkan basin.
This research is supported by Science and Engineering Research Board (grant no. SERB/CRG/2021/006505). Mathews George Gilbert received support from the Council of Scientific and Industrial Research (CSIR), New Delhi, India, under a Junior Research Fellowship (grant no. 09/087(1005)/2019-EMR-I).
This paper was edited by Frank Zwaan and reviewed by Yatheesh Vadakkeyakath and one anonymous referee.
Bhattacharya, G. C. and Yatheesh, V.: Plate-tectonic evolution of the deep ocean basins adjoining the western continental margin of India – a proposed model for the early opening scenario, Petroleum geosciences: Indian contexts, Springer, 61 pp., https://doi.org/10.1007/978-3-319-03119-4_1, 2015. a, b, c, d, e, f, g
Bijesh, C., Kurian, P. J., Yatheesh, V., Tyagi, A., and Twinkle, D.: Morphotectonic characteristics, distribution and probable genesis of bathymetric highs off southwest coast of India, Geomorphology, 315, 33–44, 2018. a, b, c
Bonini, M., Souriot, T., Boccaletti, M., and Brun, J. P.: Successive orthogonal and oblique extension episodes in a rift zone: Laboratory experiments with application to the Ethiopian Rift, Tectonics, 16, 347–362, 1997. a, b
Chaubey, A., Dyment, J., Bhattacharya, G. C., Royer, J.-Y., Srinivas, K., and Yatheesh, V.: Paleogene magnetic isochrons and palaeo-propagators in the Arabian and Eastern Somali basins, NW Indian Ocean, Geological Society, London, Special Publications, 195, 71–85, 2002. a
Collier, J., Sansom, V., Ishizuka, O., Taylor, R., Minshull, T., and Whitmarsh, R.: Age of Seychelles–India break-up, Earth Planet. Sc. Lett., 272, 264–277, 2008. a
Crameri, F., Shephard, G. E., and Heron, P. J.: The misuse of colour in science communication, Nat. Commun., 11, 5444, https://doi.org/10.1038/s41467-020-19160-7, 2020. a
Dewey, J.: Tectonic evolution of the India/Eurasia collision zone, Eclogae Geol. Helv., 82, 717–734, 1989. a
DGH2024: Directorate General of Hydrocarbons (DGH), Noida, India web page: Kerala Konkan Basin, https://www.dghindia.gov.in/index.php/page?pageId=67&name=Indian Geology, last access: 23 June 2023. a, b, c
Ganerød, M., Torsvik, T., Van Hinsbergen, D., Gaina, C., Corfu, F., Werner, S., Owen-Smith, T., Ashwal, L., Webb, S., and Hendriks, B.: Palaeoposition of the Seychelles microcontinent in relation to the Deccan Traps and the Plume Generation Zone in Late Cretaceous-Early Palaeogene time, Geological Society, London, Special Publications, 357, 229–252, 2011. a
GEBCO: General Bathymetric Chart of the Oceans, GEBCO Compilation Group (2020) GEBCO 2020 Grid, https://doi.org/10.5285/a29c5465-b138-234d-e053-6c86abc040b9, , 2020. a
Henza, A. A., Withjack, M. O., and Schlische, R. W.: Normal-fault development during two phases of non-coaxial extension: An experimental study, J. Struct. Geol., 32, 1656–1667, 2010. a, b
Katz, M. and Premoli, C.: India and Madagascar in Gondwanaland based on matching Precambrian lineaments, Nature, 279, 312–315, 1979. a, b
Kolla, V. and Coumes, F.: Extension of structural and tectonic trends from the Indian subcontinent into the eastern Arabian Sea, Mar. Petrol. Geol., 7, 188–196, 1990. a, b, c
Lovecchio, J. P., Rohais, S., Joseph, P., Bolatti, N. D., and Ramos, V. A.: Mesozoic rifting evolution of SW Gondwana: a poly-phased, subduction-related, extensional history responsible for basin formation along the Argentinean Atlantic margin, Earth-Sci. Rev., 203, 103138, https://doi.org/10.1016/j.earscirev.2020.103138, 2020. a
Nathaniel, D.: Hydrocarbon potential of sub-basalt Mesozoics of deepwater Kerala Basin, India, in: 10th Biennial International Conference & Exposition Kochi, Kochi, 23–24 November 2013, 7 pp., https://spgindia.org/10_biennial_form/P428.pdf (last access: 7 June 2023), 2013. a, b
Pande, K., Sheth, H. C., and Bhutani, R.: 40Ar–39Ar age of the St. Mary’s Islands volcanics, southern India: record of India–Madagascar break-up on the Indian subcontinent, Earth Planet. Sc. Lett., 193, 39–46, 2001. a
Patriat, P. and Achache, J.: India–Eurasia collision chronology has implications for crustal shortening and driving mechanism of plates, Nature, 311, 615–621, 1984. a
Péron-Pinvidic, G. and Manatschal, G.: From microcontinents to extensional allochthons: witnesses of how continents rift and break apart?, Petrol. Geosci., 16, 189–197, 2010. a, b
Sandwell, D. T., Müller, R. D., Smith, W. H., Garcia, E., and Francis, R.: New global marine gravity model from CryoSat-2 and Jason-1 reveals buried tectonic structure, Science, 346, 65–67, 2014. a, b
Shellnutt, J., Lee, T.-Y., Chiu, H.-Y., Lee, Y.-H., and Wong, J.: Evidence of Middle Jurassic magmatism within the Seychelles microcontinent: Implications for the breakup of Gondwana, Geophys. Res. Lett., 42, 10–207, 2015. a
Shellnutt, J. G., Yeh, M.-W., Suga, K., Lee, T.-Y., Lee, H.-Y., and Lin, T.-H.: Temporal and structural evolution of the Early Palaeogene rocks of the Seychelles microcontinent, Sci. Rep., 7, 179, https://doi.org/10.1038/s41598-017-00248-y, 2017. a
Shuhail, M., Yatheesh, V., Bhattacharya, G. C., Müller, R. D., Raju, K. K., and Mahender, K.: Formation and evolution of the Chain-Kairali Escarpment and the Vishnu Fracture Zone in the western Indian Ocean, J. Asian Earth Sci., 164, 307–321, 2018. a, b, c, d, e, f, g, h, i
Singh, K., Radhakrishna, M., and Pant, A.: Geophysical structure of western offshore basins of India and its Implications to the evolution of the Western Ghats, Geol. Soc. India, 70, 445–458, 2007. a
Singh, N. K. and Lal, N. K.: Geology and Petroleum Prospects of Konkan-Kerala Basin, in: Proc. 2nd Seminar on Petroleum Basins of India, KDMIPE and ONGC, vol. 2, 461–469, 1993. a, b, c, d, e
Smith, W. H. and Sandwell, D. T.: Global sea floor topography from satellite altimetry and ship depth soundings, Science, 277, 1956–1962, 1997. a, b
Storey, M., Mahoney, J. J., Saunders, A. D., Duncan, R. A., Kelley, S. P., and Coffin, M. F.: Timing of hot spot–related volcanism and the breakup of Madagascar and India, Science, 267, 852–855, 1995. a, b
Subrahmanyam, C. and Chand, S.: Evolution of the passive continental margins of India – a geophysical appraisal, Gondwana Res., 10, 167–178, 2006. a
Subrahmanyam, V., Krishna, K., Murthy, G., Rao, D. G., Ramana, M., and Rao, M. G.: Structural interpretation of the Konkan basin, southwestern continental margin of India, based on magnetic and bathymetric data, Geo-Mar. Lett., 14, 10–18, 1994. a, b
Torsvik, T. H., Amundsen, H., Hartz, E. H., Corfu, F., Kusznir, N., Gaina, C., Doubrovine, P. V., Steinberger, B., Ashwal, L. D., and Jamtveit, B.: A Precambrian microcontinent in the Indian Ocean, Nat. Geosci., 6, 223–227, 2013. a, b, c
Treloar, P. J. and Coward, M. P.: Indian Plate motion and shape: constraints on the geometry of the Himalayan orogen, Tectonophysics, 191, 189–198, 1991. a
Unnikrishnan, P.: Crustal structure, tectonic and sedimentation history along the Kerala-Konkan, basin, western continental margin of India based on integrated geophysical studies, PhD thesis, IIT Bombay, Mumbai, India, 2018. a
Unnikrishnan, P., Radhakrishna, M., and Prasad, G. K.: Crustal structure and sedimentation history over the Alleppey platform, southwest continental margin of India: Constraints from multichannel seismic and gravity data, Geosci. Front., 9, 549–558, 2018. a, b
Unnikrishnan, P., Gilbert, M., and Radhakrishna, M.: Crustal structure along the Kerala-Konkan Basin, southwest continental margin of India, using multi-channel seismic and gravity modelling: Implications on India-Madagascar rifting and basin evolution, J. Asian Earth Sci., 242, 105504, https://doi.org/10.1016/j.jseaes.2022.105504, 2023. a, b, c, d, e, f
Yatheesh, V., Bhattacharya, G. C., and Mahender, K.: The terrace like feature in the mid-continental slope region off Trivandrum and a plausible model for India–Madagascar juxtaposition in immediate pre-drift scenario, Gondwana Res., 10, 179–185, 2006. a
Yatheesh, V., Kurian, P. J., Bhattacharya, G. C., and Rajan, S.: Morphotectonic architecture of an India–Madagascar breakup related anomalous submarine terrace complex on the southwest continental margin of India, Mar. Petrol. Geol., 46, 304–318, 2013. a, b
Zwaan, F., Chenin, P., Erratt, D., Manatschal, G., and Schreurs, G.: Complex rift patterns, a result of interacting crustal and mantle weaknesses, or multiphase rifting? Insights from analogue models, Solid Earth, 12, 1473–1495, https://doi.org/10.5194/se-12-1473-2021, 2021. a, b